Automation in process control 1st Module
Process control block diagram
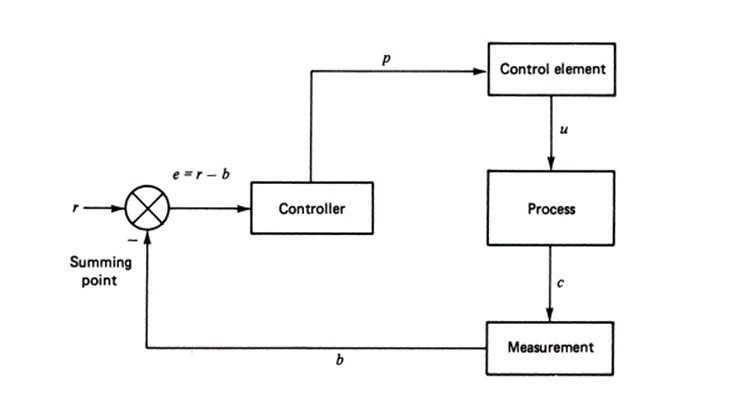
The elements of a process-control system are defined in terms of separate functional parts of the system. The following paragraphs define the basic elements of a process-control system
Figure shows a general block diagram constructed from the elements defined previously. The controlled variable in the process is denoted by c in this diagram, and the measured representation of the controlled variable is labeled b. The controlled variable setpoint is labeled r, for reference. The controller uses the error input to determine an appropriate output signal, p, which is provided as input to the control element. The control element operates on the process by changing the value of the controlling process variable, u
Process In general, a process can consist of a complex assembly of phenomena that relate to some manufacturing sequence. Many variables may be involved in such a process, and it may be desirable to control all these variables at the same time.
There are single-variable processes, in which only one variable is to be controlled, as well as multivariable processes, in which many variables, perhaps interrelated, may require regulation.
The process is often also called the plant.
Measurement
Clearly, to effect control of a variable in a process, we must have information about the variable itself. Such information is found by measuring the variable. In general, a measurement refers to the conversion of the variable into some corresponding analog of the variable, such as a pneumatic pressure, an electrical voltage or current, or a digitally encoded signal.
A sensor is a device that performs the initial measurement and energy conversion of a variable into analogous digital, electrical, or pneumatic information. Further transformation or signal conditioning may be required to complete the measurement function.
The result of the measurement is a representation of the variable value in some form required by the other elements in the process-control operation.
Error Detector The error detector is a subtracting-summing point that outputs an error signal, e = r - b, to the controller for comparison and action. This error has both magnitude and polarity. This error determination must be made before any contact action can be taken by the controller.
Although the error detector is often a physical part of the controller device, it is important to keep a clear distinction between the two
Controller The next step in the process-control sequence is to examine the error and determine what action, if any, should be taken. This part of the control system has many names, such as compensator or filter, but controller is the most common.
The evaluation may be performed by an operator (as in the previous example), by electronic signal processing, by pneumatic signal processing, or by a computer. In modern control systems, the operations of the controller are typically performed by microprocessor-based computers.
The controller requires an input of both a measured indication of the controlled variable and a representation of the reference value of the variable, expressed in the same terms as the measured value. The reference value of the variable, you will recall, is referred to as the setpoint. Evaluation consists of determining the action required to drive the controlled variable to the setpoint value.
Control Element The final element in the process-control operation is the device that exerts a direct influence on the process; that is, it provides those required changes in the controlled variable to bring it to the setpoint.
This element accepts an input from the controller, which is then transformed into some proportional operation performed on the process. This element is also referred to as the final control element.
Often an intermediate operation is required between the controller output and the final control element. This operation is referred to as an actuator because it uses the controller signal to actuate the final control element. The actuator translates the small energy signal of the controller into a larger energy action on the process
The Loop Notice in Figure that the signal flow forms a complete circuit from process through measurement, error detector, controller, and final control element. This is called a loop, and in general we speak of a process-control loop. In most cases, it is called a feedback loop, because we determine an error and feedback a correction to the process.
Control system evolution.
The application of Automatic control system is believed to be in use even from the ancient civilizations. Several types of water clocks were designed and implemented to measure the time accurately from the third century BC, by Greeks and Arabs.
But the first automatic system is considered as the Watts Fly ball Governor in 1788, which started the industrial revolution. The mathematical modelling of Governor is analysed by Maxwell in 1868.
In the 19th century, Leonhard Euler, Pierre Simon Laplace, and Joseph Fourier developed different methods for mathematical modelling. The second system is considered as Al Butz’s Damper Flapper – a thermostat in 1885. He started the company now named as Honeywell.
The beginning of the 20th century is known as the golden age of control engineering. During this time classical control methods were developed at the Bell Laboratory by Hendrik Wade Bode and Harry Nyquist.
Automatic controllers for steering ships were developed by Minorsky, Russian American Mathematician. He also introduced the concept of Integral and Derivative Control in the 1920s.
Meanwhile, the concept of stability was put forward by Nyquist and followed by Evans. The transforms were applied in control systems by Oliver Heaviside.
Modern Control Methods were developed after the 1950s by Rudolf Kalman, to overcome the limitation of classical Methods. PLC’s were introduced in 1975.
Final control:
The function of the final control element is to translate low-energy control signals into a level of action commensurate with the process under control. This can be considered an amplification of the control signal, although in many cases the signal is also converted into an entirely different form
Introduction to final control operation
Final control element operations involve the steps necessary to convert the control signal (generated by a process controller) into proportional action on the process itself. Thus, to use a typical 4- to 20-mA control signal to vary a large flow rate from, say, to certainly requires some intermediate operations.
The specific intermediate operations vary considerably, depending on the process-control design, but certain generalizations can be made regarding the steps leading from the control signal to the final control element.
For a typical process-control application, the conversion of a process-controller signal to a control function can be represented by the steps shown in Figure 1. The input control signal may take many forms, including an electric current, a digital signal, or pneumatic pressure.
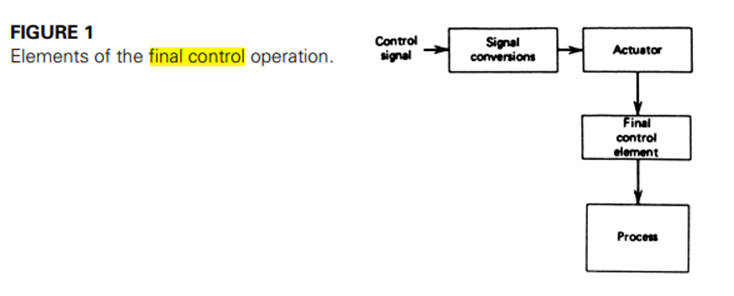
Signal conversions
This step refers to the modifications that must be made to the control signal to properly interface with the next stage of control—that is, the actuator. Thus, if a valve control element is to be operated by an electric motor actuator, then a 4- to 20-mA dc control signal must be modified to operate the motor. If a dc motor is used, modification might be current-to voltage conversion and amplification.
The devices that perform such signal conversions are often called transducers because they convert control signals from one form to another, such as current to pressure, current to voltage, and the like. A corollary to the signal-conversion process is the development of special electronic devices that provide a high-energy output under the control of a low-energy input.
This is generally described by the term industrial electronics. Section 4 presents an overview of this important topic. Control Element at last we get to the final control element itself. This device has direct influence on the process dynamic variable and is designed as an integral part of the process.
Thus, if flow is to be controlled, then the control element, a valve, must be built directly into the flow system. Similarly, if temperature is to be controlled, then some mechanism or control element that has a direct influence on temperature must be involved in the process.
This could be a heater/cooler combination that is electrically actuated by relays or a pneumatic valve to control influx of reactants
Actuators
The results of signal conversions provide an amplified and/or converted signal designed to operate (actuate) a mechanism that changes a controlling variable in the process. The direct effect is usually implemented by something in the process, such as a valve or heater that must be operated by some device.
The actuator is a translation of the (converted) control signal into action on the control element. Thus, if a valve is to be operated, then the actuator is a device that converts the control signal into the physical action of opening or closing the valve.
Control element
At last we get to the final control element itself. This device has direct influence on the process dynamic variable and is designed as an integral part of the process. Thus, if flow is to be controlled, then the control element, a valve, must be built directly into the flow system.
Similarly, if temperature is to be controlled, then some mechanism or control element that has a direct influence on temperature must be involved in the process. This could be a heater/cooler combination that is electrically actuated by relays or a pneumatic valve to control influx of reactants
Example of a process-control system showing the final control operations.
In Figure 2, a control system is shown to control the degree of baking of, say, crackers, as determined by the cracker color. The optical measurement system produces a 4- to 20-mA conditioned signal that is an analog representation of cracker color (and, therefore, proper baking). The controller compares the measurement to a setpoint and outputs a 4- to 20-mA signal that regulates the conveyer belt feed-motor speed to adjust baking time.
The final control operation is then represented by a signal conversion that transforms the 4- to 20-mA signal into a 50- to 100-V signal as required for motor speed control. The motor itself is the actuator, and the conveyer belt assembly is the control element.
Because applications of process-control techniques in industry are as varied as the industry itself, it is impractical to consider more than a few final control techniques. By studying some examples, the reader should be prepared to analyze and understand many other techniques that arise in industry
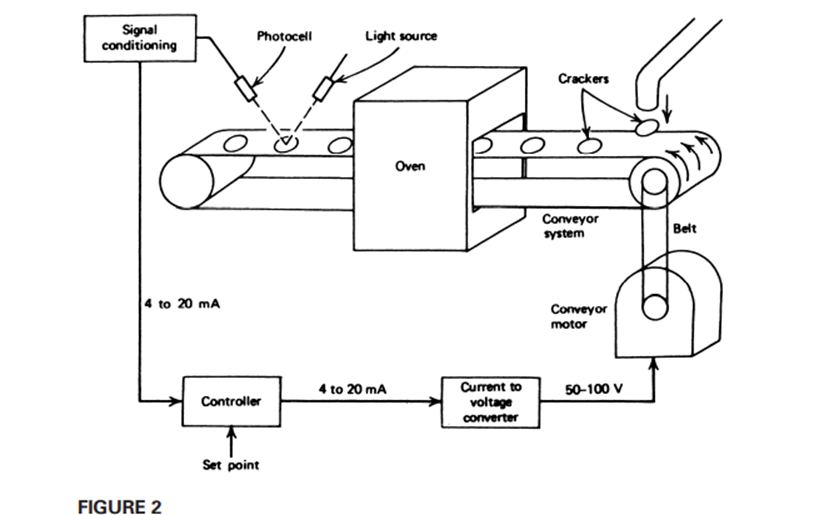
Alarm and annunciators
The purpose of an alarm system (annunciator) is to bring attention to an abnormal or unsafe operating condition in the plant. Traditional annunciators used discrete alarm modules for this purpose. These dedicated hardware units are diminished in numbers but they are used in installations where simplicity is desired or where separation from the basic process control system is required for safety reasons.
The annunciators were compact, reliable, and because of the hermetically sealed relay logic modules, they could also be mounted in certain hazardous areas in addition to the general-purpose control rooms. In order to be mounted in Class 1 explosion-proof areas, they required purging.
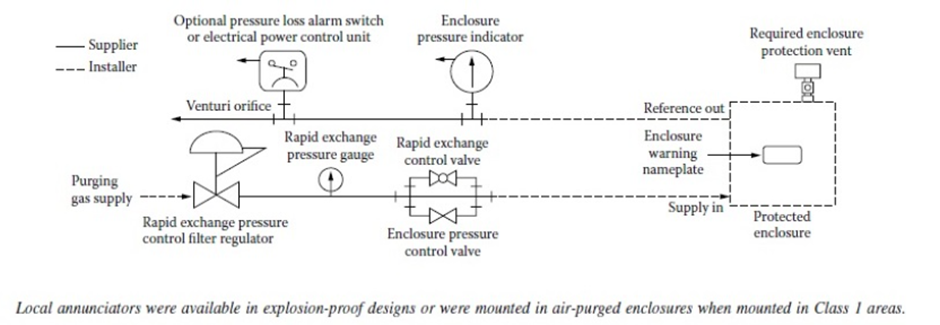
PRINCIPLES OF OPERATION
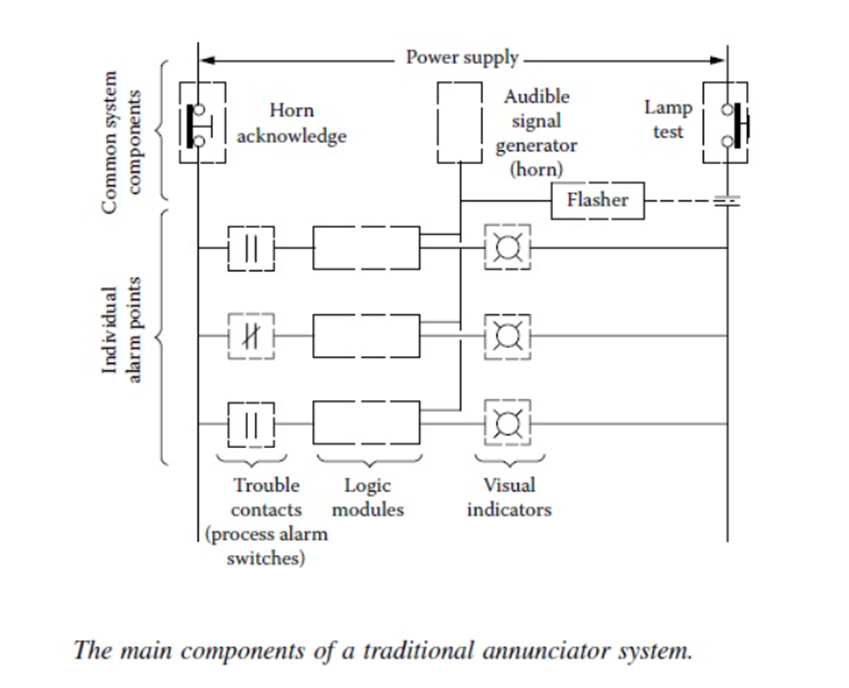
The annunciator system consists of multiple alarm points. Each alarm circuit includes a problematic contact (alarm switch), a logic module and a visual indicator. The individual alarm points are operated from a common power source and share a number of annunciator system components, including an audible signal generator (horn), a flashing light and confirmation and test buttons.
In normal operation, the annunciator system and the individual alarm points are inactive. The problem contact is an alarm switch that monitors a particular process variable and is activated when the variable exceeds the preset limits.
In electrical annunciator systems, it is usually a switch contact that closes (creates) or opens (breaks) the electrical circuit to the logic module and thereby initiates the alarm condition. In the alert state, the annunciator turns on the visual indicator of the particular alarm point, the audible signal and the flashing light for the system.
The visual indicator is usually a plate with the backlight mark engraved with an inscription to identify the variable and the abnormal condition, but it can also be a bull’s-eye with an identification plate. The audible signal can be a horn, a bell or a bell.
The flashing light is common to all individual alarm points and interrupts the circuit to the visual indicator when that point enters the alert condition. This causes the light to continue flashing intermittently until the abnormal condition returns to normal or the operator confirms it.
The horn confirmation button is provided with a momentary contact: when operated, the logic module circuit is changed to silence the audible signal, stop the flashing light and turn on the “stable” visual indicator.
When the abnormal condition is corrected, the problematic contact returns to normal, and the visual indicator automatically turns off. The test button of the lamp with its momentary contact tests the burnt lamps in the visual indicators.
When activated, the button closes a common circuit (bus) to each visual indicator in the annunciator system, lighting those lamps that are not lit as a result of an abnormal operating condition.
Control drawing:
An electrical schematic is a drawing that employs a standard set of symbols and definitions so that anyone who knows the standards can understand the operation of the circuit. In just the same way, process control employs a standard set of symbols and definitions to represent a plant and its associated control systems.
This standard was developed and approved by a collaboration between the American National Standards Institute (ANSI) and the Instrumentation, Systems, and Automation (ISA) society. The standard is designated ANSI/ISA S5.1-1984 (R1992) Instrumentation Symbols and Identification
P & ID symbols and diagrams,
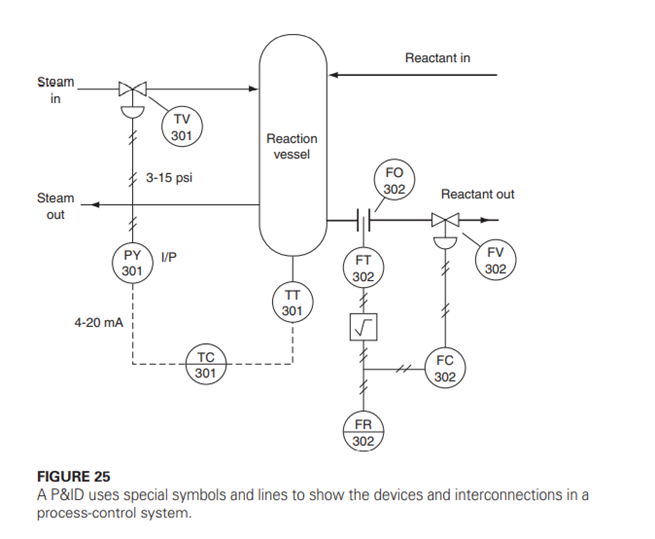
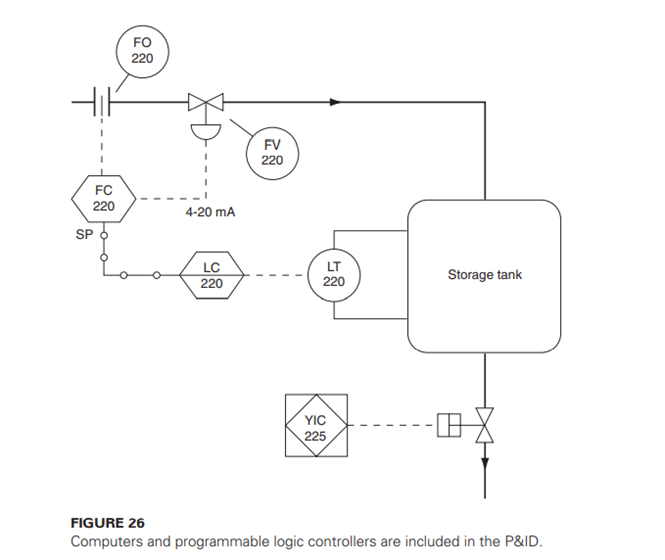
Essential Elements The P&ID depicts the entire plant and associated control systems. This includes plant operating units, product flow lines, measurement and control signal lines, sensors, controllers, final control elements, computers, and programmable logic controllers (PLCs).
Also included are letter and number designations to identify the function of an element and notes to further explain features of the P&ID.
Figure 25 shows a section of a plant with a reaction vessel into and out of which a reactant (heavy line) flows. The vessel is heated by steam input. Temperature within the vessel is controlled by controlling the steam input, and a flow control system regulates the reactant flow out of the vessel.
Figure 26 shows a system in which the level in a tank is controlled using a cascade control system. In a cascade control system, the set point of one loop is the controller output of another loop. The system in Figure 26 uses computers to provide the controller operations. Again, the primary product flow is shown as a heavy line.
Instrument Lines Symbols Figure 25 shows that the standard 4- to 20-mA current signal is represented as a dashed line in the P&ID, while the pneumatic signal (e.g., 3 to 15 psi) is presented as a line with crosshatches.
Figure 26 shows electrical (current) lines and also the digital data feed from a computer as a solid line with small bubbles
Instrument Symbols The instrumentation associated with control systems varies from sensors and transmitters to controllers, computers, and PLCs. These are drawn as bubbles with or without rectangles. In general, the instrument symbol will be identified by a letter code, which denotes its function, and by a number code assigned by the designers, which may identify the loop or some region of the plant.
Figure 25 shows that the temperature control loop has a temperature sensor and transmitter designated by TT/301, which is connected to a temperature controller, TC/301. The solid line through the controller bubble means it is accessible by an operator, as in a control room panel. When the setpoint is not indicated, that means it is manually set. The controller is connected to an I/P converter designated by PY/301.
Figure 26 shows a flow loop under computer control. This flow control computer, FC 220, is located in the field (i.e., at the site of control). This is an all-electrical loop that inputs a 4- to 20-mA signal for flow measurement and outputs a 4- to 20-mA signal to the control valve, FV/220.Alevel transmitter, LT/220, provides input to a computer, LC/220, which is located in the control room. The output of this computer is the setpoint of the flow loop. Finally, a PLC, shown as a diamond within a box, YIC/225, can open or close the tank drain valve
Other Symbols Figures 25 and 26 also show the symbols for several other el ements associated with the control systems and plants. For example, the control valves are often identified in terms of what they control. In Figure 25, the steam valve is labeled TV/301 to indicate that it is for temperature control even though it is actually controlling steam flow. Both figures show an orifice plate used to measure flow through a pipe. The valve actuator shown in Figure 26 with the PLC indicates it is spring-loaded and electrically actuated.