Thermal Engineering 2nd Module
First Law of Thermodynamics:
Statement of the First law of thermodynamics,
The first law of thermodynamics states that "When a closed system executes a cyclic process, the algebraic sum of work transfer is proportional to the algebraic sum of heat transfer". Since the system at the end of a cycle experiences no net change, the algebraic sum of heat andwork during a cycle is zero.
Symbolically,
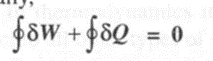
In the above equation, the symbol

designates the cyclic integraL and dow designates work and heat are inexact differentials. The above equation can also be written as
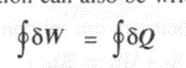

Extension of the First law to non-cyclic process,
Energy as a property ( THE FIRST LAW OF THERMODYNAMICS FOR A CHANGE IN STATE OF A CLOSED SYSTEM)
As per the definition of first law, it is applied to a closed system undergoing a cycle: But, we can apply the law for a closed system undergoing a change of state by introducing new property E.
Consider a system. Undergoing a cycle changing its state from 1 to state 2 by process A as shown in the figure 3.2
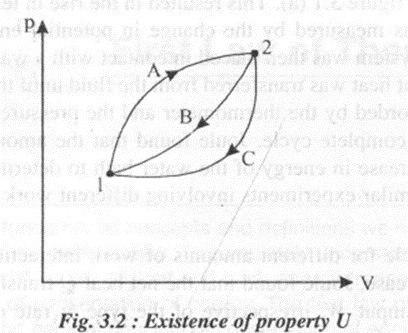
From the first law of thermodynamics,
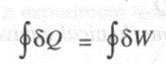
Considering two separate processes changing from state 1 to state 2 by process A and then returning to state I by process B. For this cycle we can write
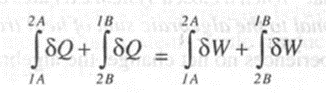
--(1)
Now consider another cycle, the system changes its state from to 2 by process A, and then returns. to state I by process C. For this cycle we can write,
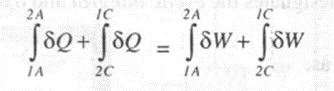
--(2)
Substituting (2) from (I), we have
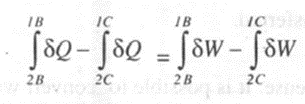
On rearranging,
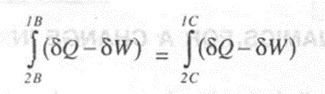
--(3)
Since Band C are two arbitrary processes between states 2 and 1, the quantity (oQ - oW) remains same for all processes between the two states.
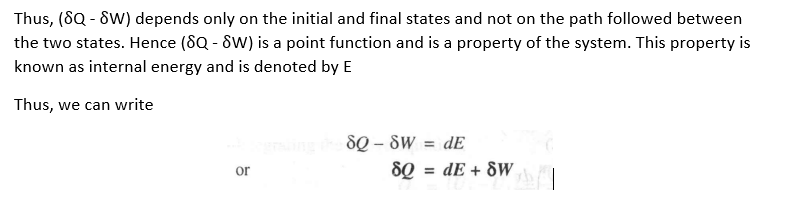

Modes of energy,( Physical significance of E)
The property E represents the sum of energy transfers across the system boundary known as stored energy. This energy might be present in a variety of forms namely, kinetic energy, potential energy, chemical energy, electrical energy, energy associated with the movement and position of the molecules etc.
However, in thermodynamics it is convenient to consider kinetic and potential energies separately and club all other types of stored energy in a single term known as internal energy U. Thus, we can write
E = Internal energy + Kinetic energy + Potential energy --- (6)
Or
E = U + KE+ PE
Since all these energies are thermodynamic properties, we can write,
dE = dU + d(KE) + d(PE) --(7)
Substituting (7) in (4), we have,

Q = dU + d(KE) + d(PE) +

W ---(8)
In the absence of motion and gravity effect, the aboveO equation reduces to,
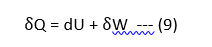
The above equation (9) is known as Non-Flow Energy Equation and is abbreviated as N.F.E.E.
Enthalpy
The sum of internal energy and the pressure –volume product consists entirely of properties which is known as Enthalpy. It is an extensive property and is denoted by symbol H
Thus H= U+pV
Pure substance
Pure substance is one which exists only in one species irrespective of phase or planes which it exists.A pure substance can exist in three different states. It is defined as the having one chemical composition or structure but capable of existing in more than one phase
Internal energy and enthalpy of pure substance
The internal energy of a pure substance can be expressed by using a two property rule. It is denoted by letter U. Internal energy is directly influenced by heat interactaions. In the absence of motion, caapalirity, gravity, electricity and magnetism, the change in internal energy of a pure substance between two states 1 and 2 can be determined as follows
From first law of thermodynamics, for unit mass of a substance,
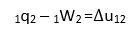
Enthalpy
Enthalpy of a pure substance is given by the sum of internal energy and pv work. Thus enthalpy is expressed as,
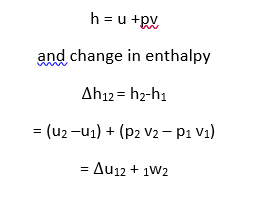
Two-property rule
Two property rule states that "The state of a pure substance of given mass can be fixed by specifying two properties provided that the system is in equilibrium with negligible effects of gravity, motion, electricity, magnetism and capilarity".
The two property rule can be applied to both liquids and gases. Since solids can have different pressures according to direction of measurement, the rule cannot be applied to them. Solids however require more than two properties to specify the state of a system. The two property rule is obeyed by some substances which are not pure.
lf a, b and c are the three properties of a system, then two property role can be symbolically represented by,
c =f (a,b)
where f() means some function of c.
Let p, v and T be the pressure, specific volume and temperature for a specific substance considered respectively
By using two property rule we can write,
pv =(A+BT)
where A and B are constants.
Specific heat at constant volume
The specific heat at constant volume is defined as the rate of change of internal energy of the system with respect to temperature when specific volume is held constant Symbolically,
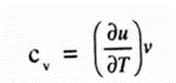
For a closed system comprising of a unit mass of pure substance from first law of thermodynamics, we have,
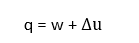
In differential form,
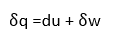
Assuming only displacement work,
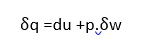
lf the volume is constant then heat transferred becomes equal to change in internal energy
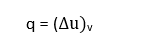
Integrating between limits
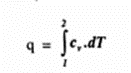
Specific heat constant pressure
The specific beat at constant pressure is defined as the rate o, change of specific enthalpy of the system with respect to temperature when the pressure is held constant.
Symbolically
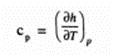
For a closed system comprising of a unit mass of pure substance, from first law of thermodynamics,
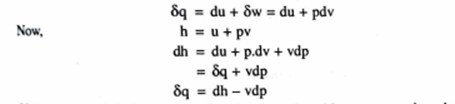
If the pressure is held constant then heat transferred becomes equal to change in enthalpy
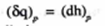
Integrating between the limits
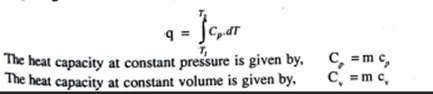
Steady state-steady flow energy equation and its important applications
Consider the flow of fluid in a control volume as shown in figure 3.10. During a small time .interval dt both mass and energy flow into and out of the control volume at sections 1-1 and 2-2 respectively
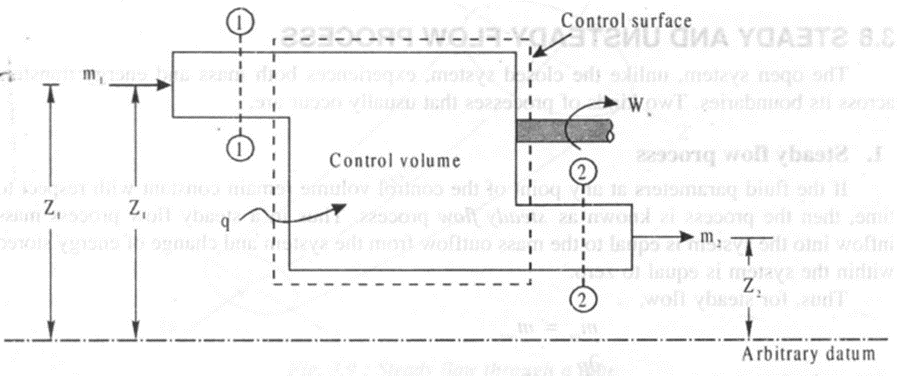

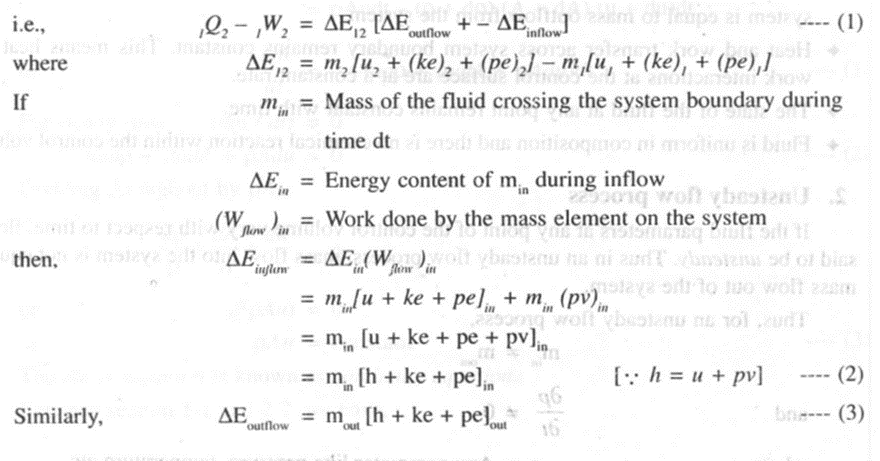
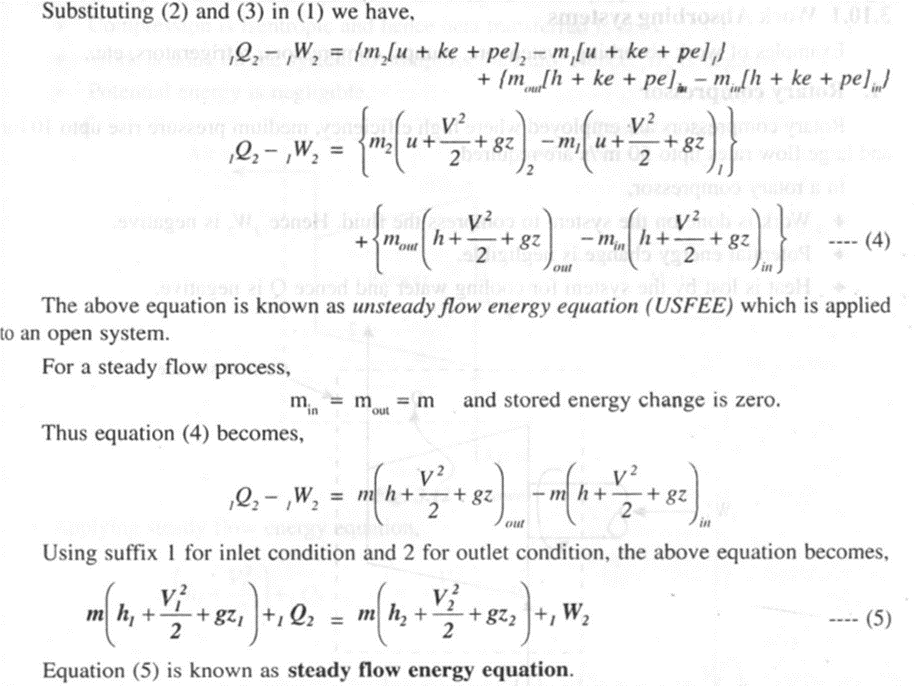
Applications
Steady flow energy equation is very useful for analysing, work developing systems, work absorbing systems and neither work developing nor work absorbing systems.
Work Absorbing systems
1.Rotary compressor
Rotary compressors are employed where high efficiency, medium pressure rise upto 10 bar and large flow rates upto 50 m)/s are required.
In a rotary compressor,
• Work is done on the system to compress the fluid. Hence W2is negative.
• Potential energy change is negligible.
• Heat is lost by the system for cooling water and hence Q is negative.
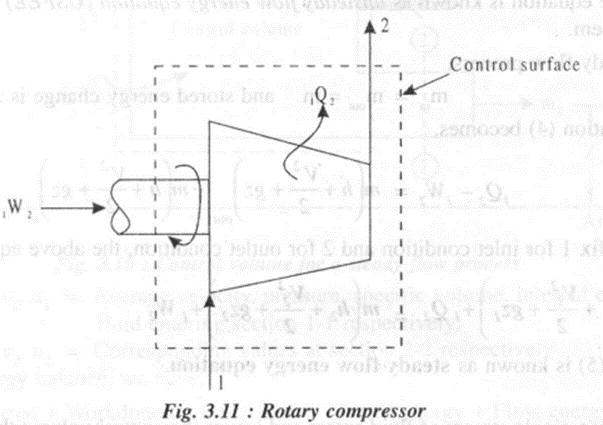
Applying steady flow energy equation,
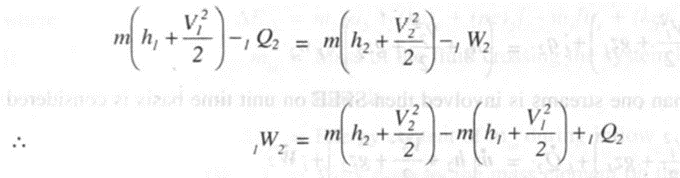
Ignoring K.E. and assuming adiabatic flow due to high flow rates,
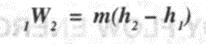
2. Reciprocating compressor
A reciprocating compressor is used to increase the pressure of the fluid as high as 1000 bar and to deliver a small quantity of the fluid (about 5 m3/s). It has a piston cylinder arrangement to compress the fluid and a receiver to ensure uniform flow rate in the control volume. In a reciprocating compressor
• Potential and kinetic energies are ignored.
• Work is done on the system to compress the fluid. Hence,W2is negative.
• Heat is lost to the system. Hence, Q2 is negative.
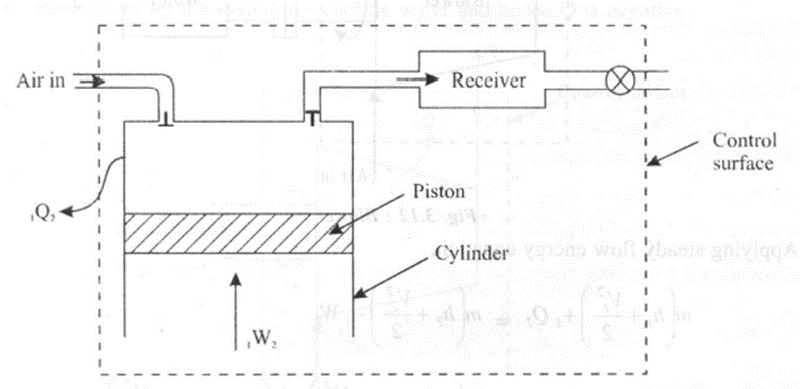
Applying steady state energy equation.

3. Centrifugal pump
A pump is a device used to convert mechanical energy into potential energy of liquid using rotating wheels called rotors. In a centrifugal pump,
• Work is done on the system which is considered as negative.
• Heat transfer is zero.
• Change in internal energy is zero due to no change in temperature of water
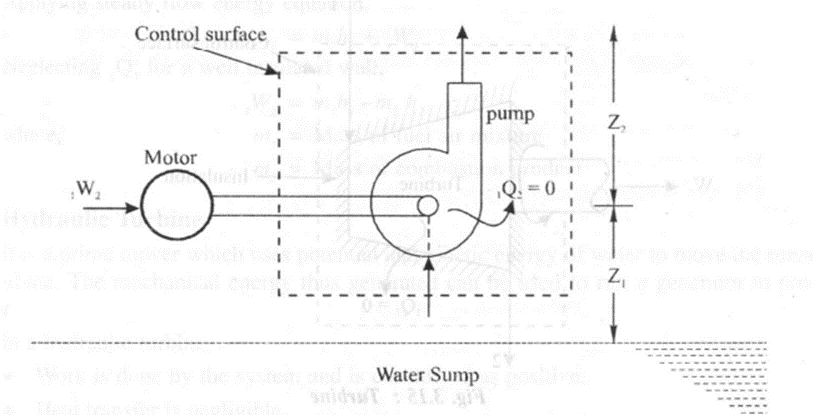
Applying steady flow energy equation,

Work developing systems
Examples of work developing system are, steam or water turbines, reciprocating engines namely IC engines and steam engines, etc.
1. Steam or Gas Turbine
A turbine is a device used to generate power using the fluid which expands from high pressure to low pressure. In a steam or gas turbine,
• Work is done by the system and hence W2 is +ve.
• Change in kinetic energy is negligible.
• Heat transfer is negligible due to insulation of walls
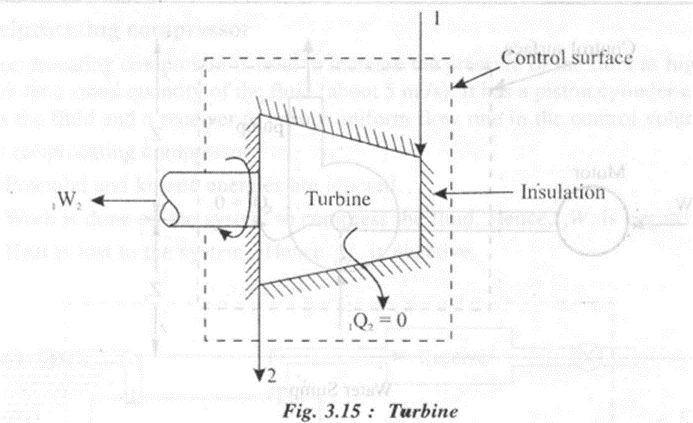
Applying steady flow energy equation.

2. Hydraulic Turbine
It is a prime mover which uses potential and kinetic energy of water to move the. Runner of the turbine. The mechanical energy thus generated can be used to run a generator to produce' power.
In a hydraulic turbine,
· Work is done by the system and it; considered as positive.
· Heat transfer is negligible.
· Change in temperature of water is zero and subsequently change in internal.energy.is neglected.
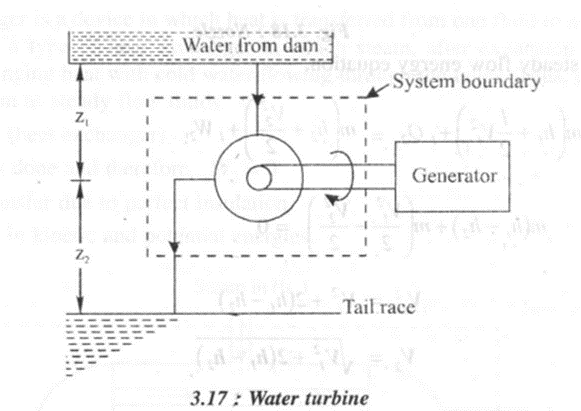
Applying steady flow energy equation,

Non work absorbing and Non work developing systems
Examples of non-work absorbing and non-work developing systems are, evaporators, boilers, all types of heat exchangers etc.
1. Heat exchanger (Condenser)
A heat exchanger is a device in which heat is transferred from one fluid to another fluid. A steam condenser is a type of heat exchanger in which steam, after expansion in the turbine condenses by exchanging heat with cold water flowing through the tubes. Thus, condenser uses both water and steam as steady flow fluids.
In a condenser (heat exchanger)
• No work is done and therefore, W2 = 0
• No heat transfer due to perfect insulation.
• No change in kinetic and potential energies.
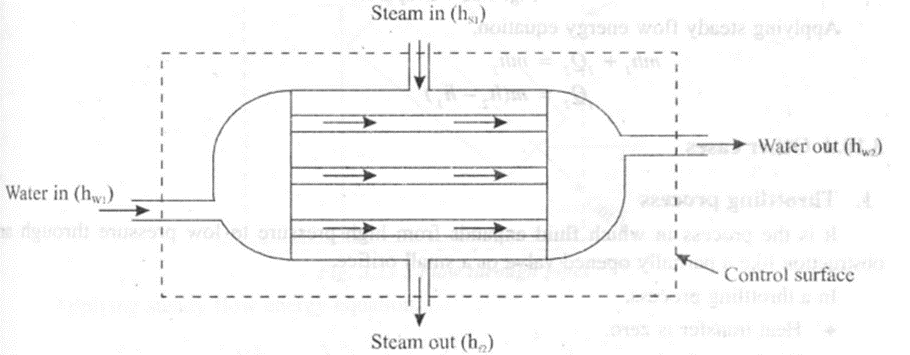
If mw and ms are the mass of water and mass of steam, hs and hr are enthalpy of steam and condensate and hwis the enthalpy of water,
then Heat lost by steam = Heat gained by water

2. Evaporator
An evaporator extracts heat from the cooling cabinet of a refrigeration system. The refrigerating liquid enters the evaporator and absorbs latent heat from the cooling cabinet and leaves the cabinet in the form of a vapour, at constant pressure.
In an evaporator,
• No work is done and therefore, W2 = 0 .
• No change in kinetic and potential energy.
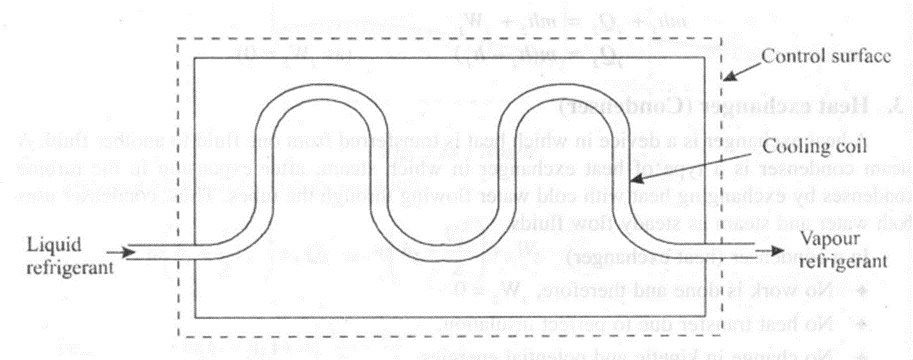
Applying steady flow energy equation,
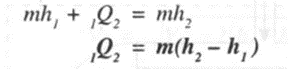
Second Law of Thermodynamics:
Thermal Reservoir
A thermal energy reservoir (TER) is a large body of i;lfinite heat capacity, capable of absorbing or rejecting heat of unlimited quantity without undergoing any appreciable changes in its thermodynamic coordinates. Any changes that take place due to heat interaction may be very slow and very minute so that all the process in a TER can be regarded as quasi-static. Consider a cyclic heat engine (CHE) as shown in fig. 4.2
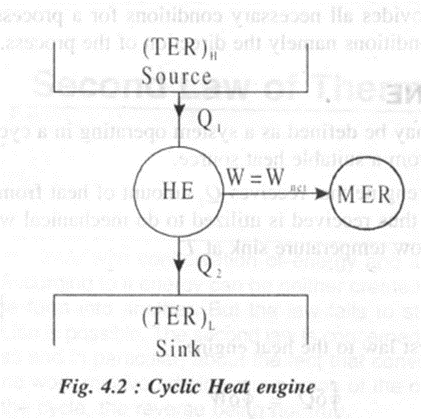
With reference to the above figure, the thermal energy reservoir (TER)Hfrom which Q1 quantity of heat is transferred to the system operating in a heat engine cycle is called source. Similarly, the thermal energy reservoir (TER)Lto which Q2 quantity of heat is rejected is called sink.
A mechanical energy reservoir (MER) is a large body essentially enclosed by an adiabatic impermeable wall capable of storing work either as potential energy or as kinetic energy. All the processes in a MER are essentially quasi-static.
Concepts of Heat Engine, Heat Pump, coefficients of performance.
HEAT ENGINE
A heat engine may be defined as a system operating in a cycle and producing useful work by abstracting heat from a suitable heat source. Consider a heat engine that receives Q1 amount of heat from a high temperature source at Tr Some of the heat thus received is utilized to do mechanical work W. The engine rejects Q2 amount of heat to a low temperature sink at T2
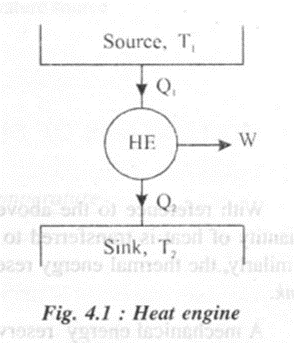
Applying the first law to the heat engine,
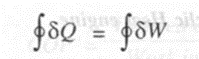
or Net heat transfer = Net work transfer
Generally the performance of the heat engine is expressed in efficiency of the engine. Thus thermal efficiency of heat engine
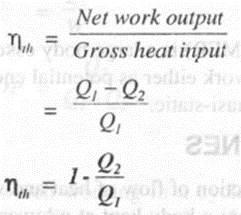
Thus, in order to increase the thermal efficiency of the heat engine we have to reduce the heat rejected to the sink Q2 or we have to increase the heat supplied to the engine Q1
Reversible heat engines,
In reversed heat engines the direction of flow of heat and work are exactly opposite. These machines are used to remove heat from a body kept at a lower temperature and to transfer this heat to a body at a higher temperature. Examples of reversed heat engines are refrigerator and heat pump.
Refrigerator
A refrigerator is a device which working in a cycle delivers heat from low temperature region to a high temperature region. The purpose of refrigerator is to cool the space by absorbing heat from it and to maintain this space at a temperature lower than that of the surroundings. Refrigerators are used to preserve food items and drugs at low temperature.
The performance of a refrigerator is expressed in terms of COP or coefficient of performance
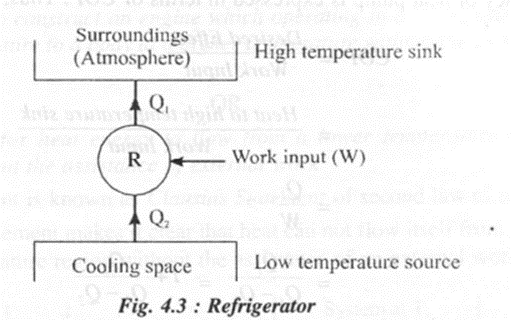
COP of refrigerator,
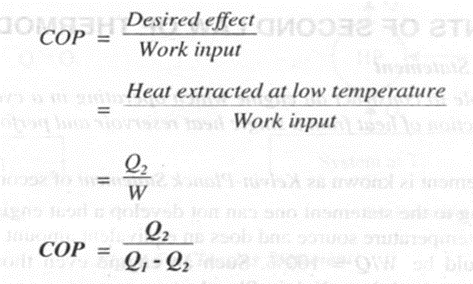
Heat Pump
A heat pump is a device which working in a cycle delivers heat from low temperature region to a high temperature region. The purpose of the heat pump is to take the heat from low temperature space and supply it to a high temperature sink for heating. Heat pumps are useful to keep the rooms warm in winter season.
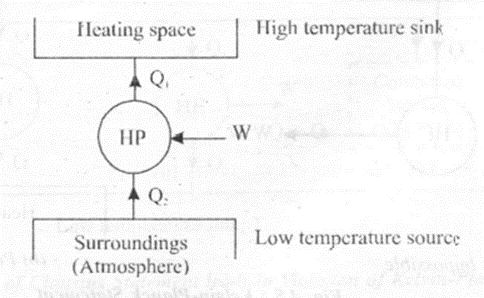
The efficiency of heat pump is expressed in terms of COP. Thus,
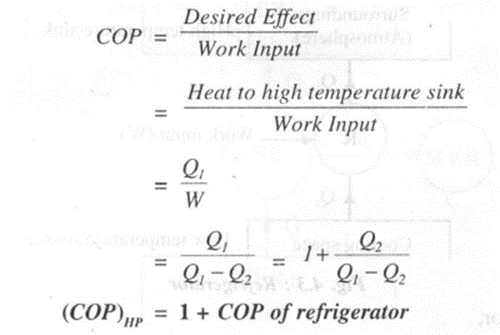
Kelvin – Planck statement of the Second law of Thermodynamics;
"It is impossible to construct an engine which operating in a cycle, will produce no other effect than the extraction of heat from a single heat reservoir and performs an equivalent amount of work".
The above statement is known as Kelvin-Planck Statement of second law of thermodynamics. Thus, according to the statement one cannot develop a heat engine that receives Q amount of heat from a high temperature source and does an equivalent amount of work W. The efficiency of such engine would be W/Q = 100%. Such an engine even though satisfies principle of conservation of energy, it violates Kelvin-Planck statement.
Thus a heat engine can receive Q1 amount of heat from a high temperature source, a part of it is utilized to do work W before Q2amount of heat is rejected to the low temperature sink as shown in figure 4.5 (b).
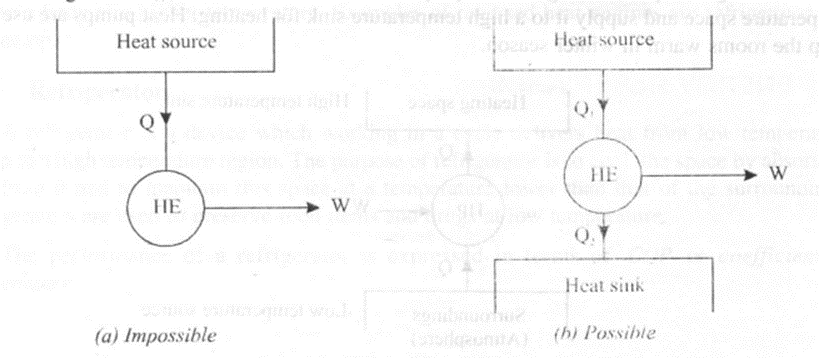
Claussius statement of second law of Thermodynamics
"It is impossible to construct an engine which operating in a cycle, transfers heat from a body at a lower temperature to a body at a higher temperature without the assistance of external work".
OR
"It is impossible for heat energy to flow from a lower temperature body to a higher temperature body without the assistance of external work ".
The above statement is known as Clausius Statement of second law of thermodynamics. Thus, Clausius statement makes it clear that heat cannot flow itself from a low temperature region to a high temperature region without the assistance of an external work.
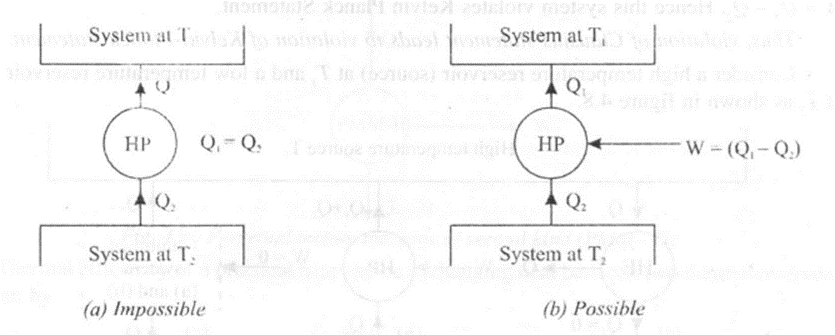
Equivalence of Kelvin Planck and Clausius statements
Consider a high temperature reservoir (source) at T1and a low temperature reservoir (sink) at T2 as shown in Figure 4.7
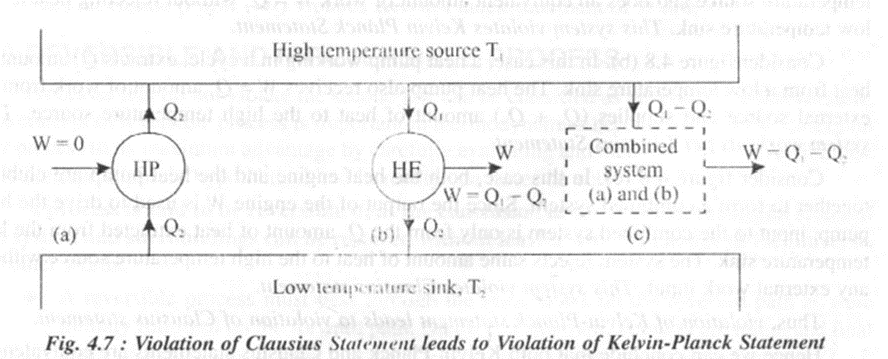
Consider figure 4.7 (a). In this case a heat pump HP operates in a cycle and transfers Q1 amount of heat from a low temperature sink to a high temperature source without any work input. This system violates Clausius statement.
Consider figure 4.7 (b). In this case, a heat engine HE operates in a cycle absorbing Q1 amount of heat from a high temperature source. The engine does W amount of work and finally rejects Q2amount of heat to the low temperature sink. This system operates as per Kelvin Planck Statement.
Consider figure 4.7 (c). In this case both the heat pump and the heat engine are combined together to form a combined system. This system constitutes a device that receives (Q1 – Q2) amount of heat from the high temperature source and does an equivalent amount of work W = Q1 – Q2 Hence this system violates Kelvin Planck Statement.
Thus, violation of Clausius statement leads to violation of Kelvin-Planck statement.
Consider a high temperature reservoir (source) at T1 anda low temperature reservoir (sink) at Tl as shown in figure 4.8.
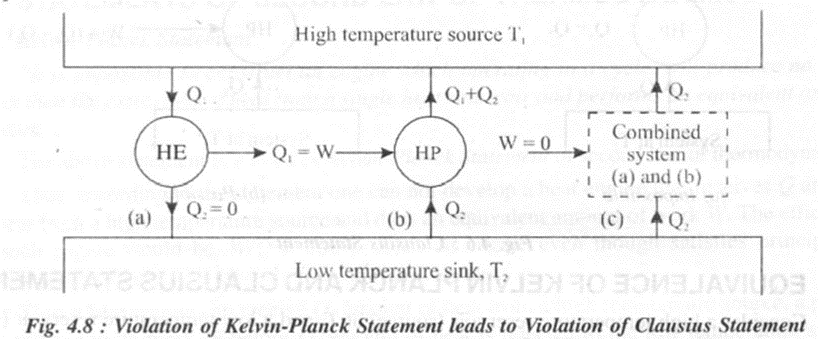
Consider figure 4.8 (a). In this case a heat engine extracts Q1 amount of heat from a high temperature source and does an equivalent amount of work W = Q1 without rejecting heat to the low temperature sink. This system violates Kelvin Planck Statement.
Consider figure 4.8 (b). In this case, a heat pump working in a cycle, extracts Q1amount of heat from a low temperature sink. The heat pump also receives W = Q1amount of work from an external source and supplies (Q1 + Q2) amount of heat to the high temperature source.This system works as per Clausius Statement.
Consider figure 4.8 (c). In this case, both the heat engine and the heat pump are clubbed together to form a combined system. Since the output of the engine W is used to drive the heat pump, input to the combined system is only from the Q2 amount of heat extracted from the low temperature sink. The system rejects same amount of heat to the high temperature source without any external work input. This system .violates Clausius statement.
Thus, violation of Kelvin-Planck statement leads to violation of Clausius statement.
Hence we can conclude that both Kelvin-Planck and Clausius statements are equivalent in sense.
Preputial motion machine of first kind
A perpetual motion machine of the first kind is an engine working in a cycle without receiving heat from any source, but delivering work to the surroundings.
From the first law of thermodynamics, we have
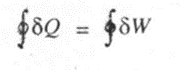
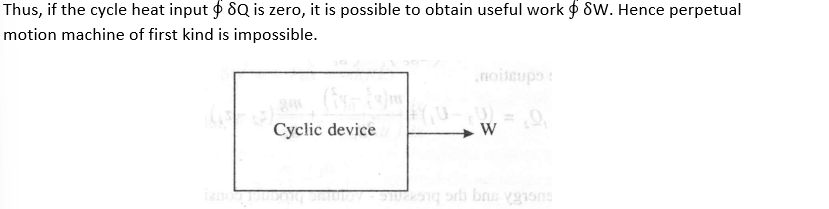

Preputial motion machine of second kind
A perpetual motion machine of second kind (PMM - If) is an engine working in a cycle developing net work by exchanging heat from a single heat source. Such an engine shown in figure 4.9 is impossible as per Kelvin-Planck statement. If such an engine were possible, it should extract .heat from an infinite heat low temperature reservoir and deliver an equivalent amount of work to the surroundings. Instead of receiving heat directly from a low temperature reservoir, if the PMM - II receives heat from a high temperature reservoir, it is possible to do work.
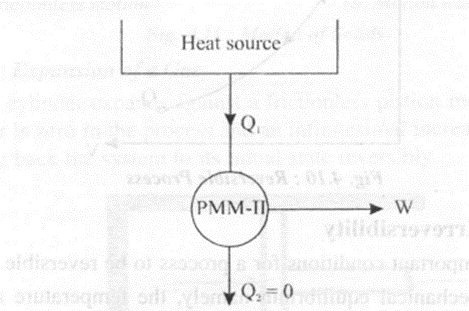
Thermal efficiency of a practical heat engine exchanging heat between two thermal reservoirs is given by
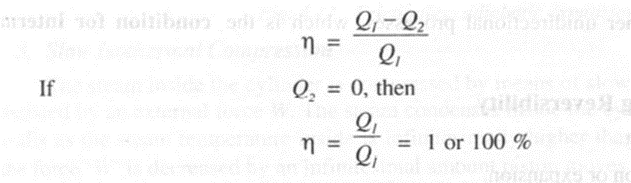
Such an engine (PMM - In violates Kelvin-Planck statement of II law
Carnot cycle, Carnot principles.
The Carnot cycle can be applied to a non-flow process with the following requirements.
· A working fluid, generally a perfect gas
· A frictionless piston sliding in a perfectly sealed cylinder. Both piston and cylinder are perfectly insulated.
· A perfectly conducting cylinder head.
· A hot source or a heat reservoir of infinite capacity at the maximum temperature T1 = Tmax
· A cold sink or a heat reservoir of infinite capacity at the minimum temperature T2 = Tmin
· All processes are reversible processes and are performed at an infinite slow pace.
As shown in the figure 4.20 on thep- V and T-s diagrams, the cycle consists of four reversible processes.

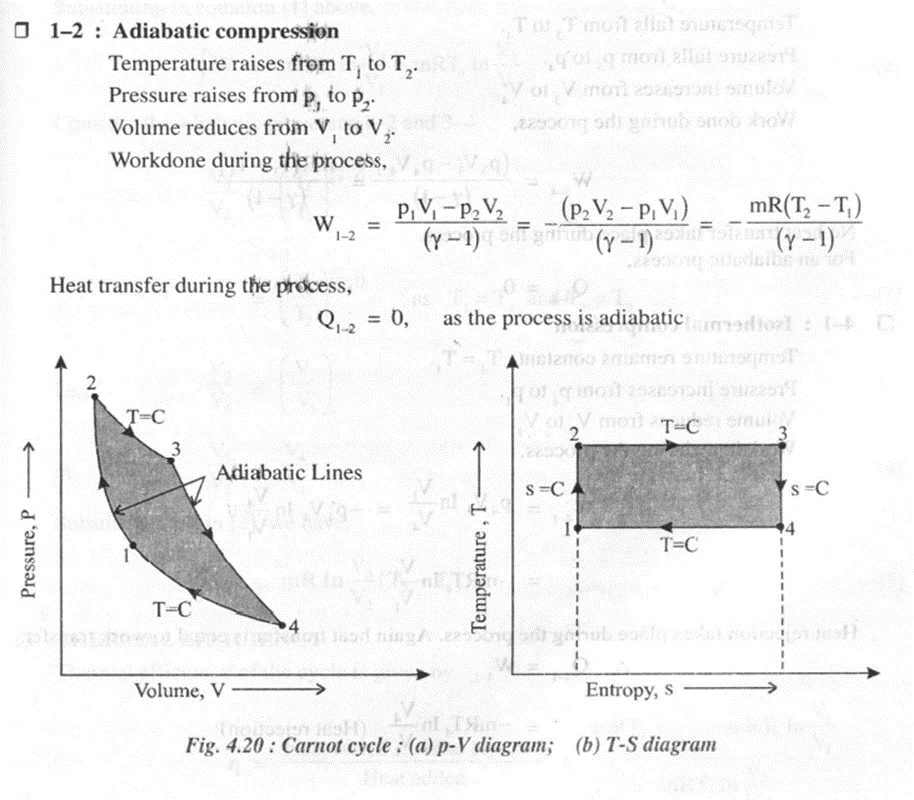
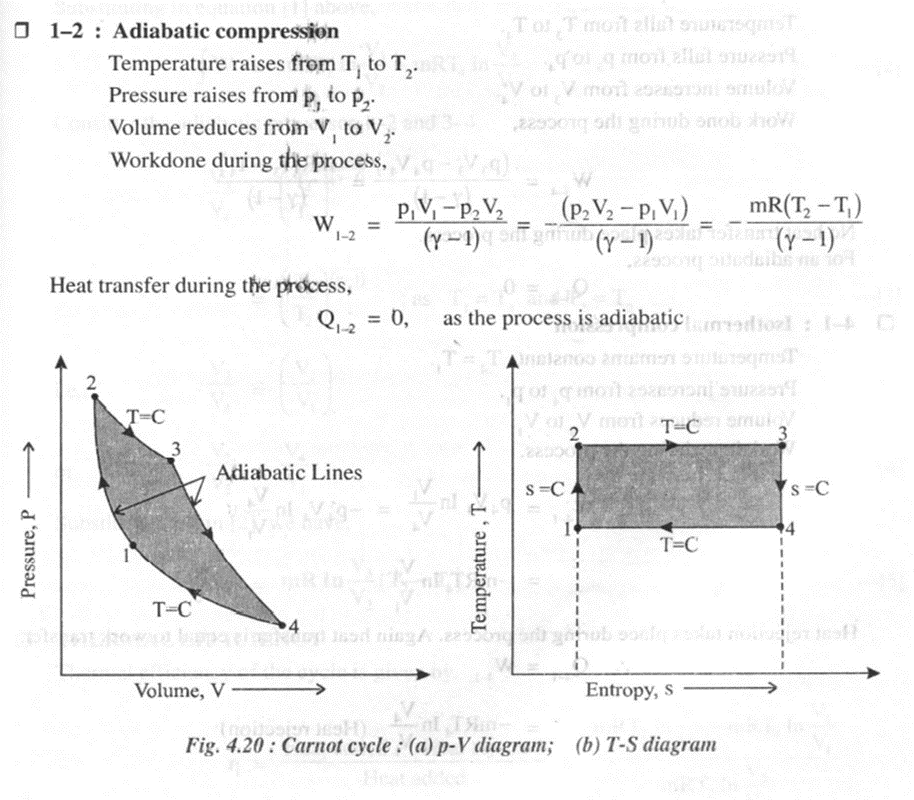
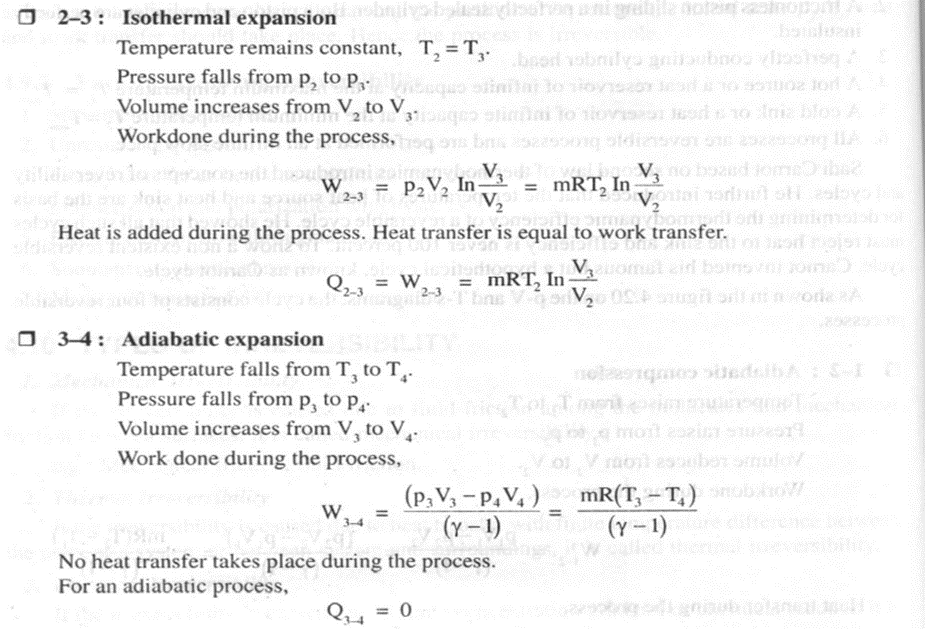
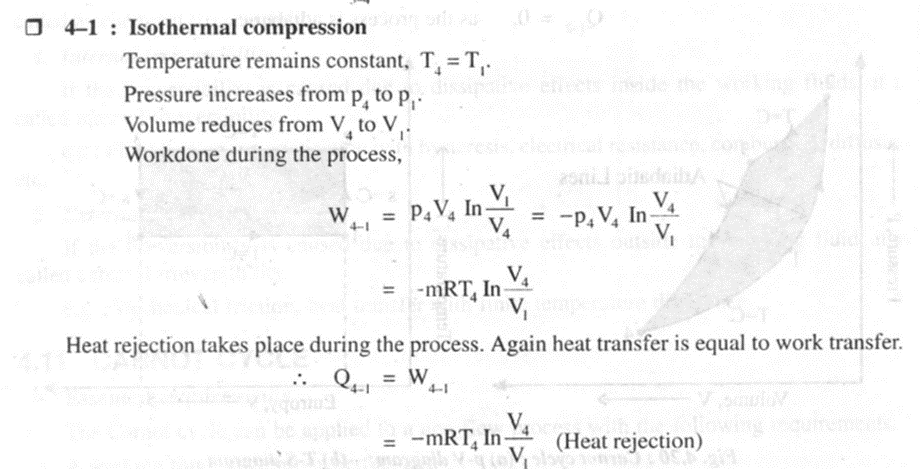
Thermodynamic temperature scale,
Any property which depends solely on temperature can be used to define a temperature scale. The thermodynamic temperature scale is the one which doesn't depend on the properties of any particular substance.
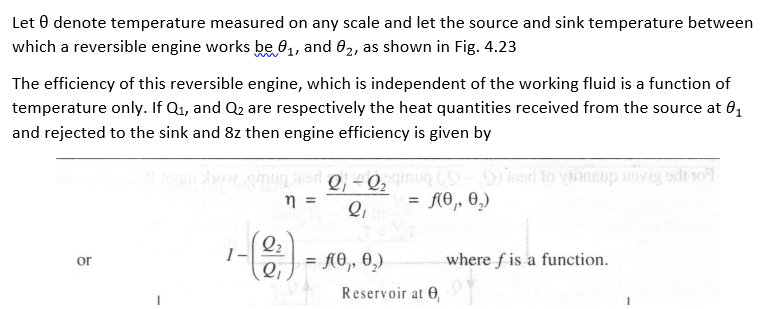
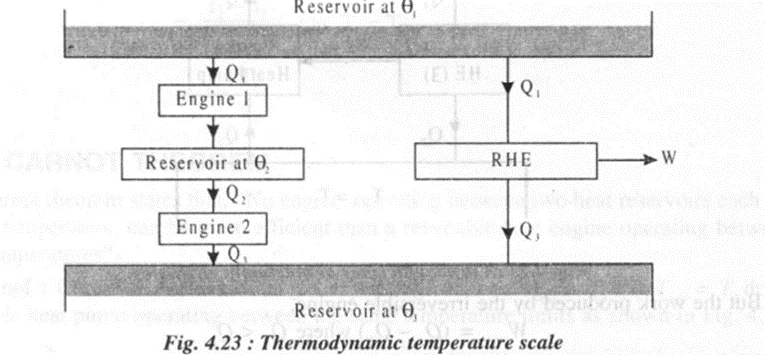
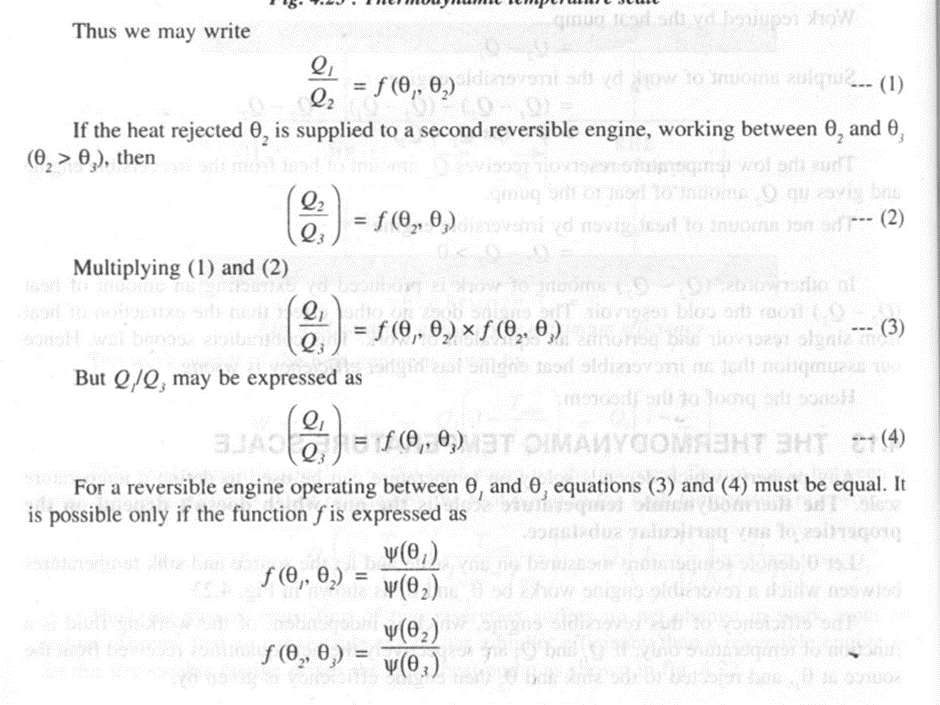
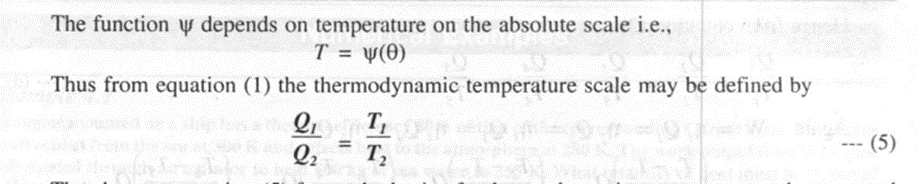
The above expression (5) forms the basis of a thermodynamic temperature scale suggested by Lord Kelvin. If one of the two temperatures is fixed and assigned a number, the other temperature can be determined. At present this fixed point or reference temperature is taken as triple point temperature of water which is assigned a numerical value of 273.16 K.
Consider a Carnot engine operating between two thermal reservoirs kept at temperatures T and Ttp respectively, Then we can write
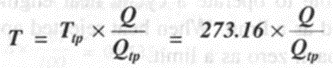
In the above equation if Q = 0, then T = 0. Thus the thermodynamic temperature scale presents the idea of absolute zero temperature.