Sensors and Signal Conditioning 3rd Module
SENSORS AND SIGNAL CONDITIONING
MODULE 3 - SELF-GENERATING SENSORS:
THERMOELECTRIC |
SENSORS, |
PIEZOELECTRIC |
SENSORS, |
PYROELECTRIC |
SENSORS, |
PHOTOVOLTAIC |
SENSORS, |
ELECTROCHEMICAL SENSORS.
Self-generating sensors yield an electric signal from a measurand without requiring any electric supply. They offer alternative methods for measuring many common quantities-in particular, temperature, force, pressure, and acceleration. Furthermore, because they are based on reversible effects, these sensors can be used as actuators to obtain nonelectric outputs from electric signals.
3.1 THERMOELECTRICSENSORS: THERMOCOUPLES
3.1.1 REVERSIBLE THERMOELECTRIC EFFECTS
Thermoelectric sensors are based on two effects that are reversible. They are the Peltier effect and the Thomson effect.
In a circuit with two dissimilar homogeneous metals A and B, having two junctions at different temperatures, an electric current arises. That is, there is a conversion from thermal to electric energy. If the circuit is opened, a thermoelectric electromotive force (emf) appears that depends only on the metals and on the junction temperatures.
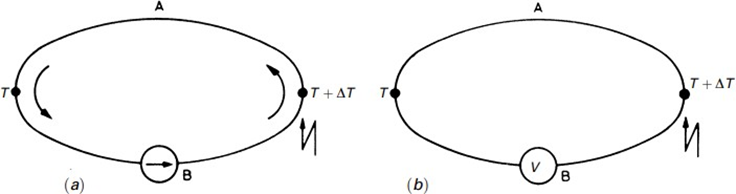
Seebeck effect in a thermocouple: (a) a current or (b) a potential difference appears when there are two metal junctions at different temperatures.
Pair of different metals with a fixed junction at a point or zone is called a thermocouple.
The relationship between the emf EAB and the difference in temperature between both junctions T defines the Seebeck coefficient SAB,
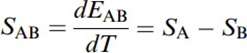
where SA and SB are, respectively, the absolute thermoelectric power for A and B. SAB depends on T, usually increasing with T. It is important to realize that while the currentflowing in the circuit dependson conductors' resistances, the emf does not depend on the resistivity, on the conductors'cross sections, or on temperature distribution or gradient. It depends only on the difference in temperature between both junctions and on the metals, provided that they are homogeneous. This emf is due to the Peltier and Thomsoneffects.
3.1.2 PELTIRE AND THOMSONEFFECTS
PELTIERâS EFFECT
The Peltier effect, named to honor Jean C. A. Peltier, who discovered it in 1834, is the heating or cooling of a junctionof two different metals when an electriccurrent flows throughit . When the currentdirection reverses, so does the heat flow. That is, if a junction heats (liberates heat), and if the current is reversed, it cools (absorbs heat), and if it cools, and when the current is reversed, it heats. This effect is reversible and does not depend on. When there is a current along a thermocouple circuit, one junction cools and the other warms. The emf dependsonly on the junction composition and temperature. Furthermore, this dependence is linear and is described by the Peltier coefficient ĎAB, sometimes called Peltier voltage because its unit is volts. ĎAB is defined as the heat generated at the junction between A and B for each unit of (positive charge) flowing from B to A; that is,

THOMSONâS EFFECT
The Thomson effect,discovered by WilliamThomson in 1847-1854, consists of heat absorption or liberation in a homogeneous conductor with a nonhomogeneous temperature when there is a current along it, as shown in figure. The heat liberated is proportional to the current, not to its square, and therefore changes its sign for a reversed current. Heat is absorbed when charges flow from the colder to the hotter points, and it is liberated when they flow from the hotter to the colder one. In other words, heat is absorbed when charge and heat flow in opposite directions, and heat is liberated when they flow in the same direction.
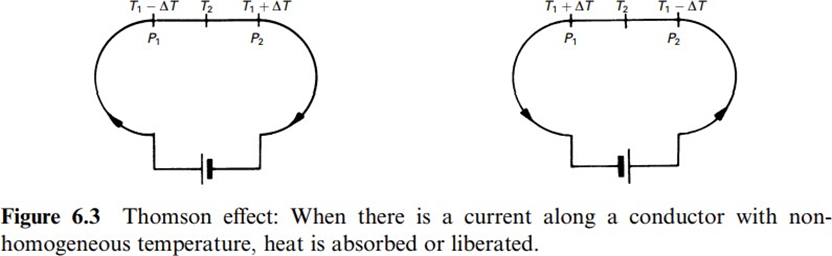
The heat flux per unit volume q in a conductor of resistivity r with a longitudinal temperature gradient dT=dx, along whichthere is a currentdensity i, is
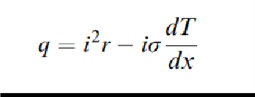
where Ď is the Thomson coefficient. The first term on the right side describes the irreversible Joule effect, and the second term describes the reversible Thomson effect
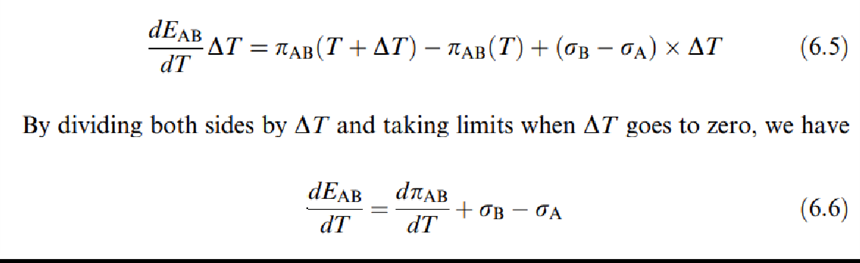
This equation constitutes the basic theorem for thermoelectricity and shows that the Seebeck effect results from the Peltier and Thomson effects.
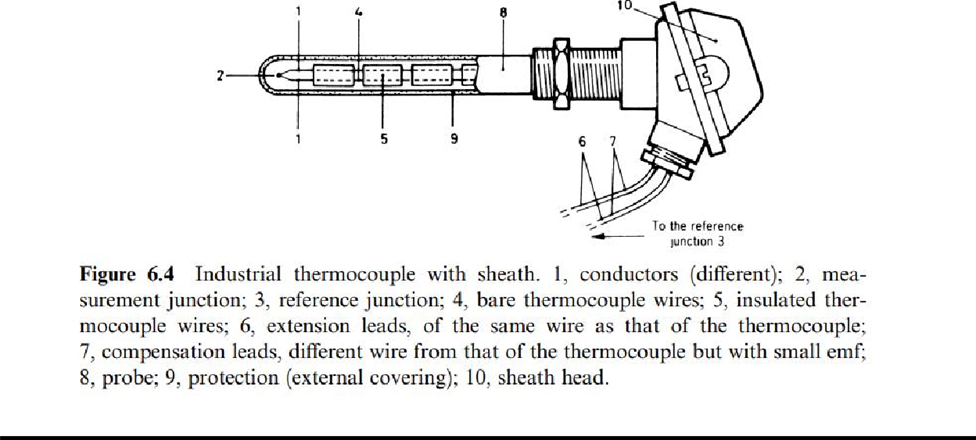
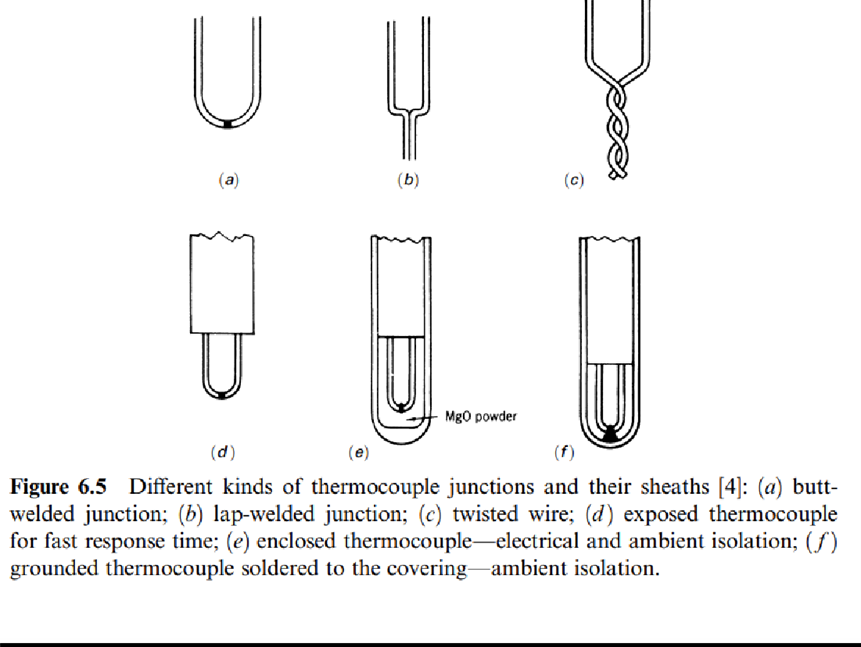
Figure 6.5 shows different junction types available. Exposed junctions are used for static measurements or in noncorrosive gas flows where a fast response time is required.
Grounded junctions suit the measurement of static temperatures or temperatures in flowing corrosive gases or liquids. They are also used in measurements performed under high pressures. The junction is soldered to the protective sheath so that the thermal responsewill be faster than when insulated.
3.1.2 PRACTICAL THERMOCOUPLE LAWS
1. Â Â LAW OF HOMOGENOUS CIRCUITS
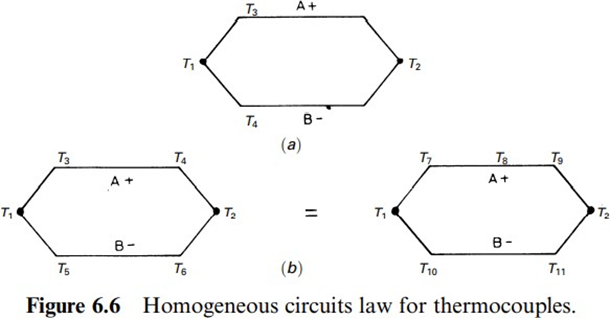
It is not possible to maintain a thermoelectric current in a circuit formed by a single homogeneous metal by only applying heat, not even by changing the cross section of the conductor. Figure 6.6 describes the meaning of this law.
In Figure 6.6a the temperatures T3 and T4 do not alter the emf due to T1 and T2. That is, if T1 & T2 and A or B are heated, there is no current. In other words, intermediate temperatures along a conductor do not alterthe emf producedby a given temperature difference betweenjunctions.
2. Â Â LAW OF INTERMEDIATE METALS
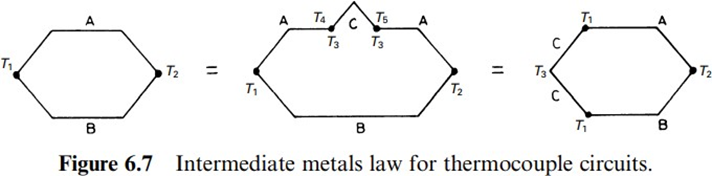
The algebraic sum of all emfs in a circuit composed by several different metals remains zero as long as the entire circuit is at a uniform temperature. This implies that a meter can be inserted into the circuit without addingany errors, provided that the new junctions inserted are allat the same temperature, as
indicated in Figure 6.7. The measuring instrument can be inserted at a point in a conductor or at a junction. Nichrome is used in wire wound resistors and strain gages. CuO/Cu yields a large emf, it is advisable to keep electric contacts clean. A analogy of this law is that if the thermal relationship between each of two materials and a third one is known, then it is possible to deduce the relationship between the two first ones. Therefore, it is not necessary to calibrate all the possible metal pairs in order to know the temperature corresponding to a given emf measured with a given pair. Rather, its behaviorwith respect a third materialis enough. The reference metal isplatinum.
3. Â Â LAW OF SUCCESSIVE OR INTERMEDIATE TEMPERATURES
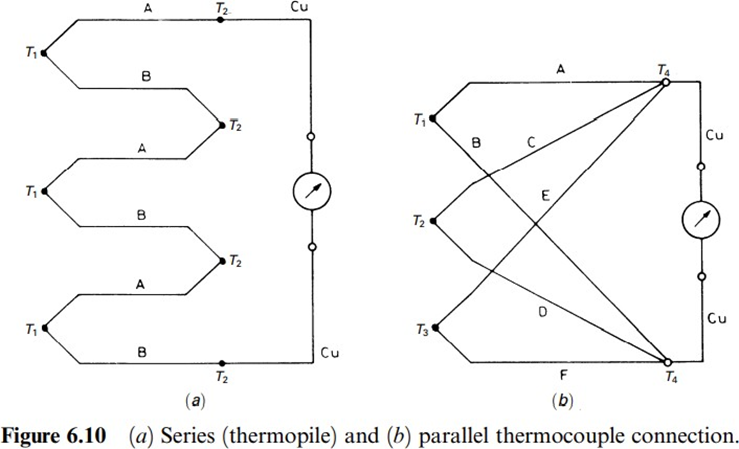
If two homogeneous metals yield an emf E1 when their junctions are at T1 and T2, and an emf E2 whenthey are at T2 and T3, then the emf when the junctions are at T1 and T3 will be E1 + E2 (Figure 6.9). This means, for example, that it is not necessary for the reference junction to be at 0 C. Any other reference temperature is alsoacceptable.
The previous laws enable us to analyze circuits such as those in Figure 6.10. Case (a) shows several thermocouples connected in series, thus constituting a thermopile. It is straightforward to verify that this increases the sensitivity compared to the case where a single thermocouple is used. Case (b) shows a parallel connection, which yields the average temperature if all thermocouples are linear in the measurement range and have the same resistance.
3.2 Â PIEZOELECTRIC SENSORS
3.2.1 THE PIEZOELECTRIC EFFECT
Piezoelectricity is the electric chargethat accumulates in certain solidmaterialsâsuch as crystals, certain ceramics, and biological matter such as bone, DNA, and variousproteinsâin response to applied mechanical stress. The word piezoelectricity means electricity resulting from pressure and latent heat.
Therefore, when applying an electric voltage between two sides of a piezoelectric material, it strains. Piezoelectricity must not be confused with ferroelectricity, whichis the property of having a spontaneous or induced electric dipole moment. All ferroelectric materials are piezoelectric, but the opposite is not always true. Piezoelectricity is related to the crystalline (ionic) structure. Ferromagnetism is instead related to electron spin. Piezoelectric equationsdescribe the relationship between electric and mechanical quantities in a piezoelectric material. In Figure 6.14a, where two metal plates have been placed to form a capacitor, for a dielectric nonpiezoelectric material we have that an appliedforce F yieldsa strain S that, according to Hooke's law, in the elastic range is
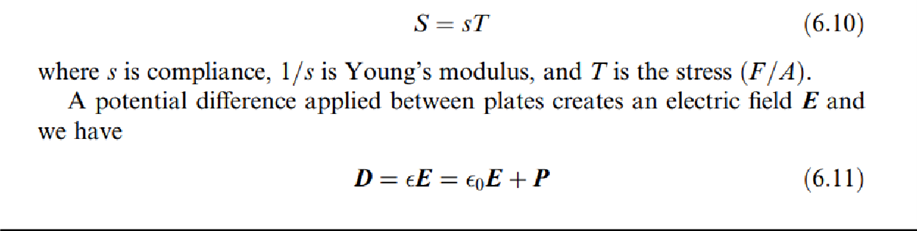
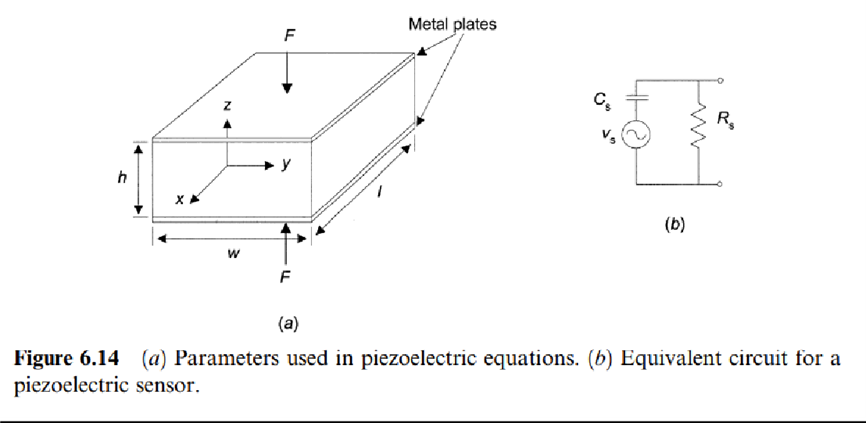
where D is the displacement vector (or electric flux density), Ń is the dielectric constant, ŃO =8:85 pF/m is the permittivity of vacuum, and P is the polarization vector.
3.2.2 PIEZOELECTRIC MATERIALS
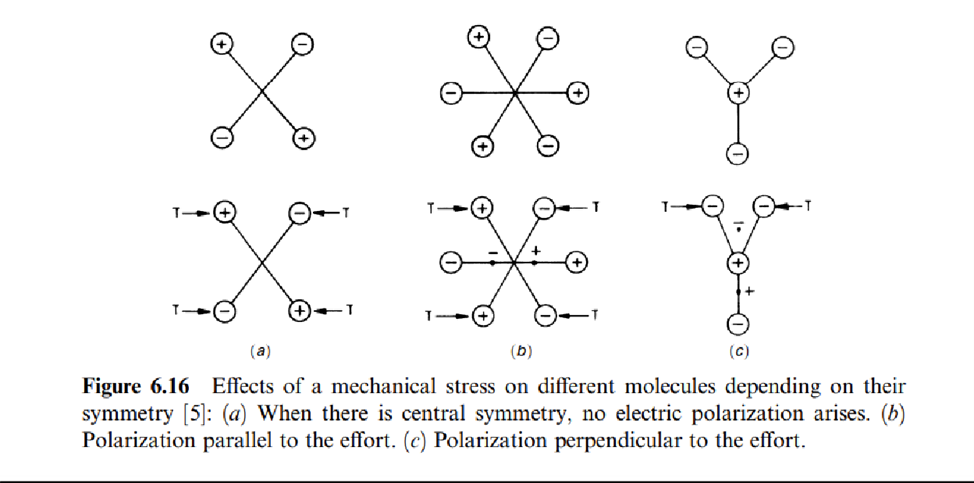
Piezoelectric properties are present in 20 of the 32 crystallographic classes, although only a few of them are used; they are also present in amorphous ferroelectric materials.
Of those 20 classes, only 10 display ferroelectric properties. All piezoelectric materials are necessarily anisotropic.
In case (a) there is central symmetry. An applied force does not yield any electric polarization. In case (b), on the contrary, an applied force yields a parallel electric polarization, while in case (c) an applied force yields a perpendicular polarization. The natural piezoelectric materials most frequently used are quartz and tourmaline. The synthetic materialsmore extensively used are not crystalline but ceramics.
Piezoelectric ceramics display a high thermal and physical stability and can be manufactured in many different shapes and with a broad range of values for the properties of interest. Their main disadvantages are the temperature sensitivity of their parameters and their susceptibility to aging (loss of piezoelectric properties) when they are close to their Curie temperature. The most commonly used ceramics are lead zirconate titanate (PZT ), barium titanate, and lead niobate. Bimorphs consist of two ceramicplates glued together and with opposite polarization.
Some polymers lacking central symmetry also display piezoelectric properties with a value high enough to consider them for those applications where because of the size and shape required it would be impossible to use other solid materials. The most common is polyvinylidene fluoride (PVF2 or PVDF ), whose piezoelectric voltage coefficient is about four times that of quartz. In order to improve the mechanical properties for piezoelectric sensors, piezoelectric ``composite'' materials are used. They are heterogeneous systems consisting of two or more different phases, one of which at least shows piezoelectric properties.
3.2.3 Â APPLICATIONS
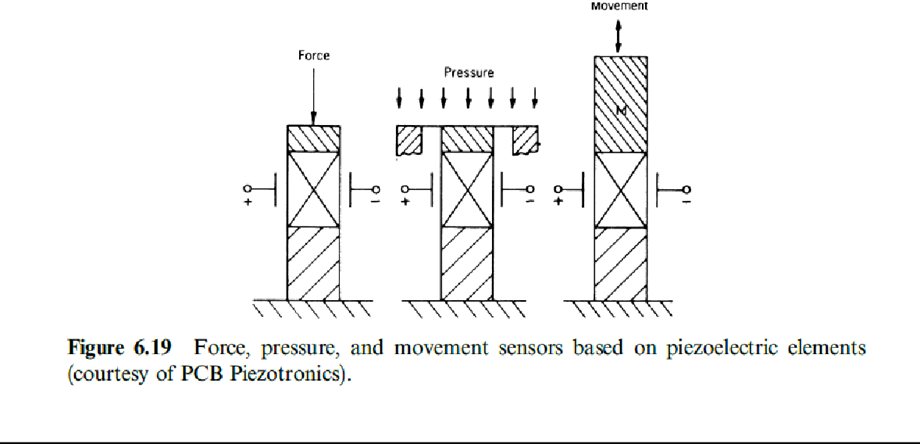
1. Figure 6.19 shows an outline for the three types of sensors. This similarity makes these sensors sensitive
to the three quantities, and therefore special designs are required that minimize interference.
2. Pulse measurement - they are sensitive enoughto record pulse measurements and can be
bonded to the patientâs body with self-adhesive.
3. Stethoscopes - because of their high sensitivity and robustness piezo sensors are often used withinstethoscopes.
4. Anesthesia Effectiveness - piezo sensors are used to measure the effectiveness of anesthesia as theymeasure the response of muscles to electrical stimulation.
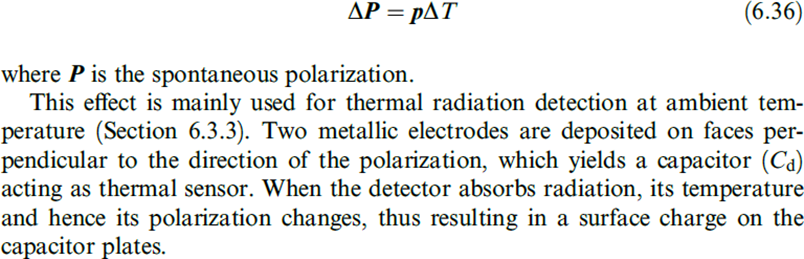
3.3 Â PYROELECTRIC SENSORS
3.3.1 THE PYROELECTRIC EFFECT
The pyroelectric effect is analogous to the piezoelectric effect, but instead of change in stress displacingelectric charge, now it refers to change in temperature causing change in spontaneous polarization and resulting changein electric charge.
When the change in temperature DT is uniform throughout the material, the pyroelectric effect can be described by means of the pyroelectric coefficient, which is a vector p with the equation.
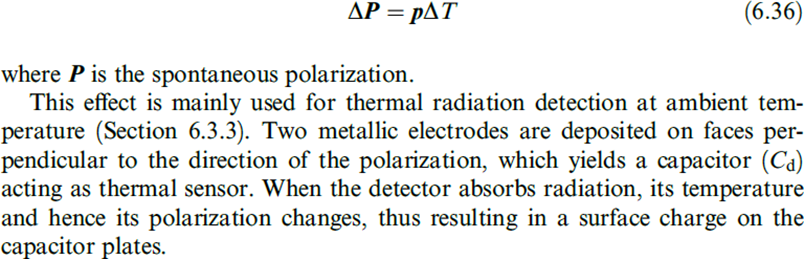
3.3.2 PYROELECTRIC MATERIALS
Pyroelectricity, like piezoelectricity, is also based on crystal anisotropy, many of the piezoelectric materials are also pyroelectric. Ten of the 21 non-Centro symmetrical crystallographic classes have a polar axis ofsymmetry. All of them displaypyroelectric properties.
There are two groups of pyroelectric materials: linear and ferroelectric. The polarization of linear materials cannot be changed by inverting the electric field.This group includesmaterials such as tourmaline, lithiumsulfate, and cadmiumand selenium sulfides.Some ferroelectric materials with pyroelectric properties are lithium tantalate, strontium and barium niobate, lead zirconate-titanate, and triglycine sulfate (TGS). Some polymeric materials such as polyvinylidene (PVF2 or PVDF ) are also pyroelectric. Pyroelectric properties disappear at the Curie temperature.
3.3.3 Â THE RADIATION LAWS: PLANK, WIENANDSTEFAN-BOLTZMAN
Any body at a temperature greater than 0 K radiates an amount of electromagnetic energy that dependson its temperature and physicalproperties. At temperatures above 500 C, the emittedradiation is visible.Below 500 C, including ambient temperatures, infrared radiation predominates so that only heat energy is perceived.
We give the name ``blackbody'' to a theoretical body that absorbs all the energy incident on it (thereby increasing its temperature). A closed space with black walls and controlled temperature, and where only a small aperture is provided, behaves approximately as a blackbody.
The ratio between the energy emitted by a given body per unit area per unit time and that emitted by ablackbody under the same conditions is the emissivity of that body E. For a blackbody, E = 1. The emissivity depends on the wavelength, the temperature, the physical state, and the chemical characteristics of the surface.
The energy WĆ emitted by the blackbody per unit time, per unit area, at a given wavelength Ć and temperature T, is given by Planck's law
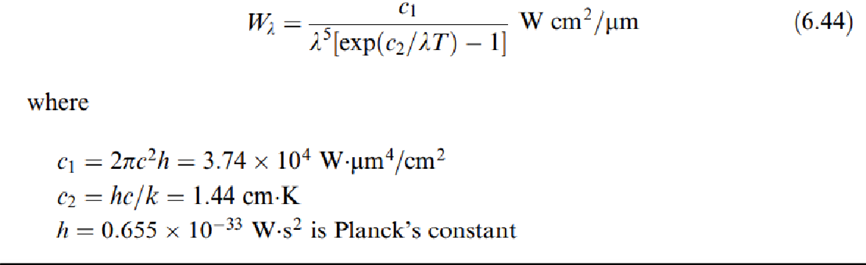
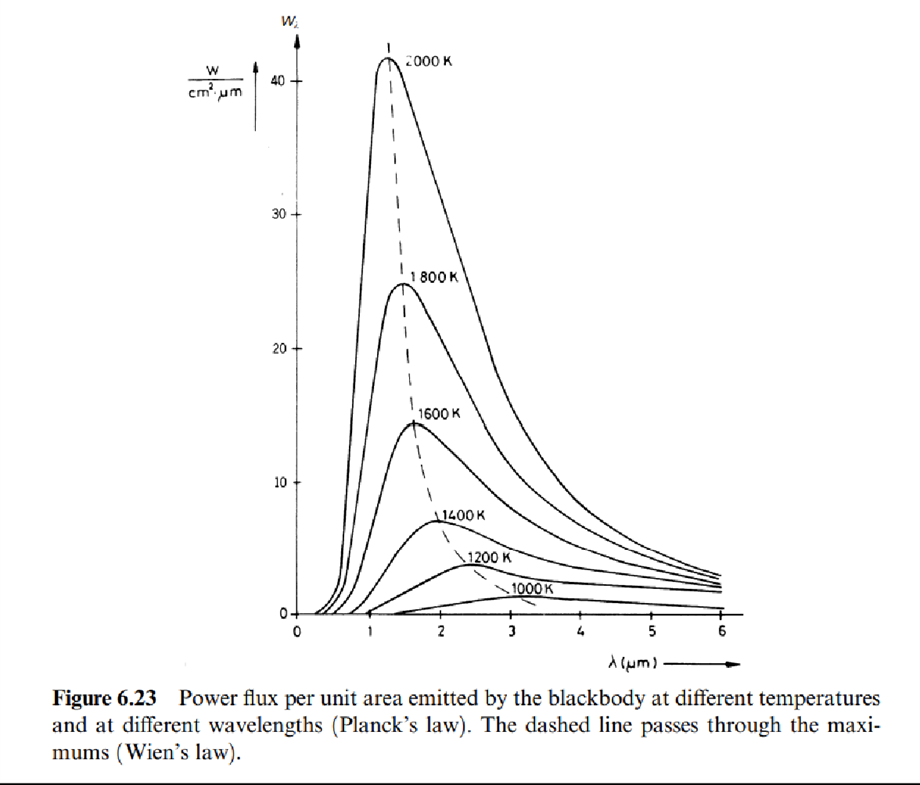

which is the equation for Wien's displacement law (to honor the man who discovered it before Planck's law was discovered). It indicates that the maximum is obtained at a wavelength that decreases for increasing temperatures.
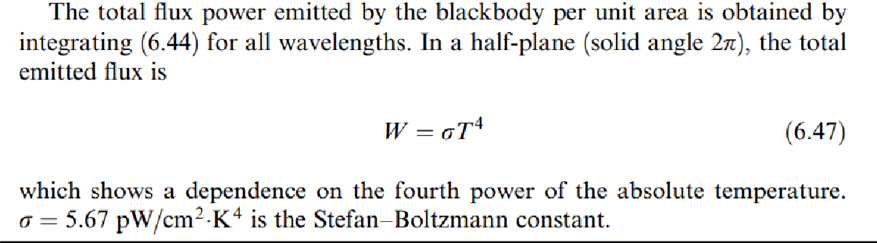
Stefan-Boltzmann law, statement that the total radiant heat power emitted from a surface is proportional to the fourth power of its absolute temperature.
3.3.4 APPLICATIONS
The most commonapplication for the pyroelectric effect isthe detection of thermal radiation at ambient temperature. It has been applied to pyrometers (noncontact temperature meters in furnaces, melted glass or metal, films, and heat loss assessment in buildings).
Other applications are IR analyzers (basedon the strong absorption of IR by CO2 and other gases),intruder and position detection, automatic faucet control, fire detection, high-power laser pulse detection, and high-resolution thermometry (6 mK). Medicalthermometers that measureear temperature detect infrared radiation from the eardrumand surrounding tissue.
3.4 Â PHOTOVOLTAIC SENSORS
3.4.1 THE PHOTOVOLTAIC EFFECT
When the internal photoelectric effect occurs in a p-n junction, it is possible to obtain a voltage that is a function of the incoming radiation intensity. The photovoltaic effect is the generation of an electric potential when the radiation ionizes a region where there is a potential barrier. When a p-doped semiconductor (doped with acceptors) contacts an n-doped semiconductor (doped with donors), because of the thermal agitation there are electrons that go into the p region and ``holes'' that move into the n-region. There they recombine with charge carriers of opposite sign. As a result, at both sides of the contact surface there are very few free charge carriers. Also, the positive ions in the n region and the negative ions in the p region, fixed in their positions in the crystal structure, produce an intense electric field that opposes the diffusion of additional charge carriers through this potential barrier. This way an equilibrium is attained between the diffusion current and the current induced by this electric field. By placing an external ohmic connection on each semiconductor, no voltage difference is detected
because the internal difference in potential at the junction is exactly compensated by contact potentials in the external connections to the semiconductor. Figure 6.24 shows that radiation whose energy is larger than the semiconductor.
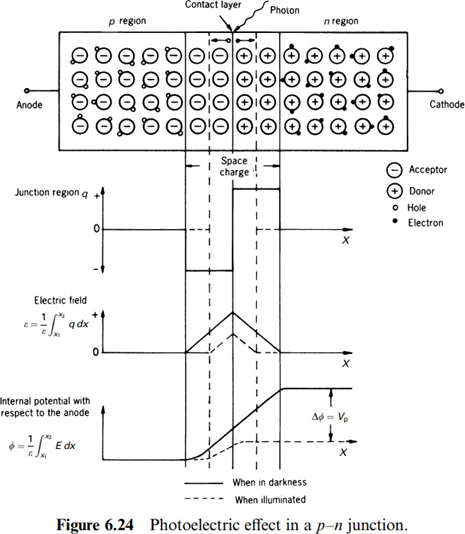
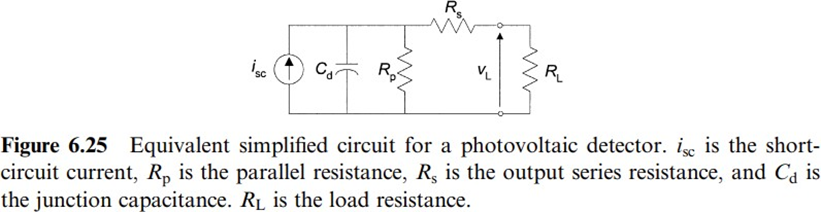
3.4.2 PHOTOVOLTAIC MATERIALS & APPLICATIONS
In addition to p-n junctions, there are other methods that produce a potential barrier, but p-n junctions are by far the most common one. If the p-n junction is between semiconductors of the same composition, then itis called a homojunction. Otherwise, it is called a heterojunction.
We select materials for the particular wavelength to be detected. In the visible and near-infrared regions, silicon and selenium are used. Silicon is in the form of homojunctions. Selenium in the form of a selenium layer ( p) covering cadmium oxide (n). For silicon sometimes an intrinsic (non-doped) silicon region is added between the p and n regions ( p-i-n detectors). This results in a wider depletion region, which yields a better efficiency at large wavelengths, faster speed, and lower noise and dark current. At other wavelengths, germanium, indium antimonide (SbIn), and indium arsenide (AsIn), among others, are used.
Photovoltaic detectors offer better linearity, are faster, and have lower noise than photoconductors, but they require amplification. For large-load resistors, the linearity decreases and the time of response increases. Photovoltaic detectors are used either in applications where light intensity is measured or in applications where light is used to sense a different quantity.
They are used, for example, in analytical instruments such as flame photometers and colorimeters, in infrared pyrometers, in pulse laser monitors, in smoke detectors, in exposure meters in photography, and in card readers. Commercial models are available consisting of a matched emitter-detector pair, some of which are alreadyconnected to a controlrelay.
3.5 Â ELECTROCHEMICAL SENSORS
Potentiometric electrochemical sensors yield an electric potential in response to a concentration change in a chemical sample. Amperometric(a chemical titration in which the measurement of the electric current flowing under an applied potential difference between two electrodes in a solution is used for detecting the end point) sensors. They are not self-generating sensors and are potentiometric sensors based on the voltage generated in the interface between phases having different concentrations. This is the same principle for voltaic cells.
Assume that there is only one ion species whose concentration changes from one phase to another, or that there are more ions but a selective membrane allows only one specific ion to go through it. Then the tendency for that ion to diffuse from the high-concentration region to the low-concentration region is opposed by an electric potential difference due to the ion electric charge. When we have equilibrium between both forces (diffusion and electric potential), the difference in potential is given by the Nernst equation is,
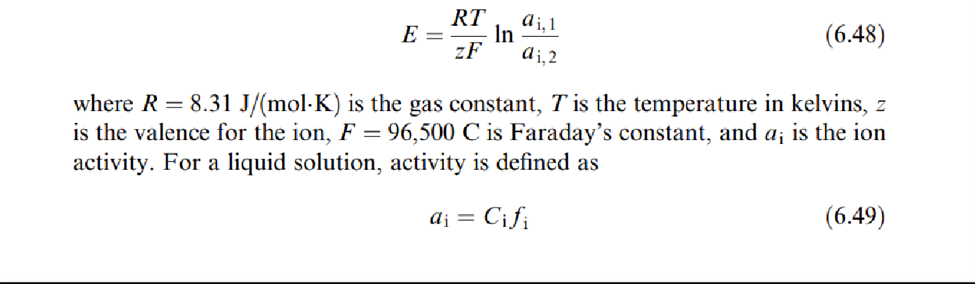
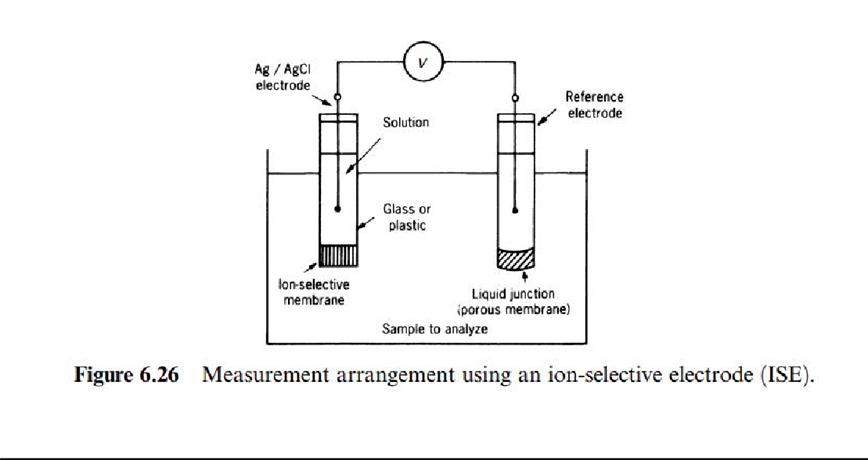
where Ci is the concentration for species i, and fi is the activity coefficient, which describes the extent towhich the behavior of species i diverges from the ideal. This measurement principle is applied by using a two electrode arrangement (Figure 6.26 ). One electrode includes the membrane that is selective to the ion of interest, and it contains a solution having a known concentration for ion species i. The other electrode is a reference, and all ions present in the sample to be measured can freely diffuse through its membrane.

Depending on the material for the membrane, there are different kinds of selective electrodes. Primary electrodes have a single membrane, which may be crystalline.
When it is crystalline, it can be homogeneous or heterogeneous. In heterogeneous electrodes the crystalline material is mixed with a matrix o inert material. Crystalline membrane electrodes are applied to concentration measurement for F-, Cl-, Br-, I-, Cu2+, Pb2+, and Cd2+, among others. The most common electrodes with a non-crystalline membrane are glass electrodes, like those used for pH andNa+ measurement.
Glass composition is chosen depending on the ion to be analyzed. Some metal salts have high electric conductivity and can be deposited on a metal electrode to act as electrolyte. These are termed solid- state electrodes.
Other electrodes use a membrane (such as PVC or polyethylene) that includes an ion exchanger or a neutral material that transports the ion. K+, for example, is measured in a PVC membrane. The most common double-membrane electrodes are gas electrodes. This method is applied, for example, to concentration measurement for CO2, SO2, andNO2.
ISEs are used for concentration measurement in multiple applications where they have often replaced flame photometers. They are used, for example, in agriculture to analyze soils and fertilizers, in biomedical sciences and clinical laboratories for blood and urine analysis, in chemical and food industries, and in environmental monitoring to measure ambient pollution.
Solid electrolyte oxygen sensors rely on the influence that oxygen ions adsorbed by a metal oxide have on the concentration of charge carriers and, hence, on conductivity of the oxide based on ions, hence it is an electrolyte.
Their main shortcomings are that they need a high temperature to work and that they have a low sensitivity to pressure changes. For this same reason, however, they can operate over a wide range of oxygen concentration. They are extensively used to determine the air-to-fuel ratio in internal combustion engines for example, in automobiles, boilers, and furnaces